
Advertisement

Can you calculate how far away lightning struck by thunder?
- Share Content on Facebook
- Share Content on LinkedIn
- Share Content on Flipboard
- Share Content on Reddit
- Share Content via Email
Sound travels through air at " the speed of sound ." Officially, the speed of sound is 331.3 meters per second (1,087 feet per second) in dry air at 0 degrees Celsius (32 degrees Fahrenheit). At a temperature like 28 degrees C (82 degrees F), the speed is 346 meters per second.
As you can see, the speed of sound changes depending on the temperature and the humidity ; but if you want a round number, then something like 350 meters per second and 1,200 feet per second are reasonable numbers to use. So sound travels 1 kilometer in roughly 3 seconds and 1 mile in roughly 5 seconds.
When you see the flash of a lightning bolt , you can start counting seconds and then divide to see how far away the lightning struck. If it takes 10 seconds for the thunder to roll in, the lightning struck about 2 miles or 3 kilometers away.
For more information on lightning and related topics, check out the links on the next page.
Lightning/Thunder FAQs
What causes thunder during lightning, what should you not do during lightning, are you safe in a car during lightning storm, should i unplug everything during a thunderstorm, lots more information, related howstuffworks articles.
- How Lightning Works
- What causes a sonic boom?
- What is relative humidity and how does it affect how I feel outside?
More Great Links
- NOAA: Thunder and Lightning
Please copy/paste the following text to properly cite this HowStuffWorks.com article:
Speed Of Sound Calculator
The speed of sound is the distance travelled per unit time by a sound wave as it propagates through an elastic medium. In dry air at 20 °C (68 °F), the speed of sound is 343.2 metres per second ( 1,126 ft/s ; 1,236 km/h ; 768 mph ; 667 kn ), or a kilometre in 2.914 s or a mile in 4.689 s.
In common everyday speech, speed of sound refers to the speed of sound waves in air. However, the speed of sound varies from substance to substance: sound travels most slowly in gases; it travels faster in liquids; and faster still in solids. For example (as noted above), sound travels at 343.2 m/s in air; it travels at 1,484 m/s in water(4.3 times as fast as in air); and at 5,120 m/s in iron. In an exceptionally stiff material such as diamond, sound travels at 12,000 m/s ; which is around the maximum speed that sound will travel under normal conditions.
The speed of sound is variable and depends on the properties of the substance through which the wave is travelling.
Humidity has a small but measurable effect on the speed of sound (causing it to increase by about 0.1%–0.6%), because oxygen and nitrogen molecules of the air are replaced by lighter molecules of water. This is a simple mixing effect.
Christmas-Why? (Creation.com)
GoodNewsPost.com (KJV Bible)
FineTunedUniverse.com
Back To AnyCalculator.com
speed of sound calculator, speed of sound, air density, air temperature, speed of sound waves in the air, sound waves, humidity, temp, air, sound, density, fahrenheit, celsius, kelvin, rankine, mph, knots, meters a second, feet per second, kilometers an hour, at the spped of sound, can you hear me now
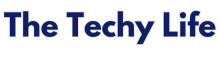
How Long Does It Take Sound to Travel 1 Mile? Explained
Sound is an integral part of our everyday lives, allowing us to communicate, perceive the world around us, and even enjoy music and entertainment. Yet, have you ever wondered how long it takes for sound to travel a certain distance? Specifically, how long does it take for sound to travel one mile? Exploring the science behind sound propagation can provide us with fascinating insights into the speed and behavior of this invisible force that shapes our auditory experiences. In this article, we will delve into the intricacies of sound travel, examine the factors that influence its speed, and offer a comprehensive explanation of the time it takes for sound to cover a mile. Get ready to embark on a journey that will undoubtedly reveal the captivating nature of sound transmission.
Table of Contents
Basics of Sound Waves
Explanation of sound as a mechanical wave.
In order to understand how long it takes sound to travel 1 mile, it is essential to have a basic understanding of sound waves. Sound is a form of mechanical wave that travels through a medium, such as air, water, or solids. It is produced when an object vibrates, causing molecules in the medium to vibrate and transfer energy from one location to another.
Unlike electromagnetic waves, which can travel through a vacuum, sound waves require a medium to propagate. This is because sound waves rely on the interaction and movement of particles within the medium to transmit energy.
Description of compression and rarefaction
As sound waves travel, they create areas of high pressure called compressions and areas of low pressure called rarefactions. These compressions and rarefactions are responsible for the characteristic patterns of sound waves.
During a compression, particles in the medium are pushed closer together, resulting in a higher density of molecules. This increase in density creates a corresponding increase in pressure. In contrast, during a rarefaction, particles in the medium become more spaced out, resulting in a lower density of molecules and a decrease in pressure.
The alternating pattern of compressions and rarefactions creates a wave-like motion that allows sound to travel. It is important to note that while the particles within the medium vibrate back and forth, the overall motion of the sound wave itself is in a forward direction, perpendicular to the direction of particle motion.
Understanding the basics of sound waves, including the concept of compression and rarefaction, is crucial for further exploration into the speed of sound and its travel time in different media.
Speed of Sound in Air
Exploration of factors affecting the speed of sound.
In this section, we will delve into the factors that influence the speed of sound in air. The speed of sound in any medium is determined by three main properties: stiffness, density, and temperature. Stiffness refers to the medium’s ability to resist deformation under stress, while density relates to how closely packed the particles of the medium are. Temperature affects the speed of sound because it affects the particles’ ability to move and transmit energy.
Demonstration of the formula for calculating sound speed
To calculate the speed of sound in air, we can use the formula v = √(γ * R * T), where v represents the speed of sound, γ is the adiabatic index (1.4 for dry air), R is the gas constant (287 J/(kg·K) for air at sea level), and T is the temperature in Kelvin. This formula allows us to determine the speed of sound at any given temperature.
The speed of sound in dry air at sea level and 20°C (293K) is approximately 343 meters per second or 767 miles per hour. However, it’s important to note that the speed of sound is not constant and can vary depending on external conditions. For example, sound travels faster in higher temperatures because the particles have more energy and can vibrate more rapidly, increasing the speed of sound.
Other factors such as humidity and atmospheric pressure can also have minor effects on the speed of sound. Increased humidity can slightly decrease the speed of sound due to the added mass of water vapor in the air. Conversely, higher atmospheric pressure can slightly increase the speed of sound by compressing the air molecules closer together and increasing their elastic properties.
Understanding the speed of sound in air is crucial in various fields, including aviation, meteorology, and even everyday activities like communication. For example, pilots need to consider the speed of sound when calculating distances and travel times during flights. Similarly, meteorologists use the speed of sound to estimate the distance of thunderstorms based on the time interval between the flash of lightning and the sound of thunder.
In conclusion, the speed of sound in air is influenced by factors such as stiffness, density, and temperature. By using the formula v = √(γ * R * T), we can calculate the speed of sound at a given temperature. However, it is important to consider other variables such as humidity and atmospheric pressure, which can have minor impacts on the speed of sound. Understanding the speed of sound in air is essential in various applications and fields.
ISpeed of Sound in Different Media
Comparing Sound Speed in Air, Water, and Solids
Sound speed varies depending on the medium through which it travels. In this section, we will compare the speed of sound in air, water, and solids, and discuss the factors affecting this variation.
How Density and Elasticity Affect Sound Speed
Density and elasticity play significant roles in determining the speed of sound in different media. In general, denser materials tend to have higher sound speeds, while more elastic materials also exhibit higher sound speeds.
Air, being a gas, has relatively low density and elasticity compared to water and solids. Consequently, sound travels at a slower speed through air, with an average value of about 343 meters per second (or roughly 767 miles per hour) at room temperature.
Water, on the other hand, has a higher density and greater compressibility than air, resulting in a higher sound speed. An average value of approximately 1482 meters per second (or around 3315 miles per hour) can be observed for sound traveling through water.
Solids, such as metals or rocks, exhibit even higher sound speeds due to their higher density and greater elasticity. The speed of sound in solids can range from a few thousand meters per second to tens of thousands of meters per second, depending on the specific material.
Factors Affecting Sound Speed in Different Media
Apart from density and elasticity, other factors also impact the speed of sound in different media. In gases, such as air, temperature plays a crucial role. As temperature increases, gas molecules move faster, leading to an increase in sound speed. Humidity, which affects the gas composition, can also have a minor influence.
In liquids, temperature and pressure are significant factors. Increased temperature causes molecules to vibrate more vigorously, resulting in faster sound propagation. High pressures can cause changes in liquid density and compressibility, affecting the speed of sound.
For solids, the arrangement and bonding of atoms within the material greatly influence sound speed. Properties like crystal structure, density, and temperature can all impact the speed at which sound waves travel through a solid medium.
In conclusion, the speed of sound varies depending on the medium through which it travels. Air has a lower sound speed compared to water, while solids exhibit even higher sound speeds. Density and elasticity are key factors determining these variations, with other factors such as temperature, pressure, and material properties also contributing to differences in sound speed. By understanding the speed of sound in different media, we can gain insights into various phenomena and applications, ranging from underwater acoustics to seismology.
Variables Affecting Sound Travel Time
Explanation of distance as a key aspect.
In the study of sound travel time, distance plays a crucial role. As sound is a form of energy that travels in waves, the time it takes for sound to travel a certain distance depends on the length of that distance. When sound waves propagate through a medium, they create alternating regions of compression and rarefaction. These oscillations determine the frequency and wavelength of the sound, which in turn affect its speed and travel time.
The distance traveled by sound is measured in units such as meters, kilometers, or miles. As these distances increase, the travel time of sound also increases. It follows a direct relationship – the greater the distance, the longer it takes for sound to traverse that distance. Understanding this relationship is crucial in various fields, such as telecommunications, where it is necessary to determine the time it takes for a signal or message to reach its destination.
Mention of other factors such as temperature and humidity
While distance is a key factor in determining sound travel time, it is not the sole contributor to variations in speed. Other factors, such as temperature and humidity, also play a role. Sound waves travel faster in warmer air because the molecules have higher kinetic energy, allowing them to vibrate more quickly. Conversely, in colder air, molecules have lower kinetic energy, resulting in slower vibrations and slower sound travel time.
Humidity, which refers to the amount of water vapor present in the air, also affects sound travel time. Moist air is denser than dry air, which causes sound waves to propagate more slowly. Therefore, higher levels of humidity result in slower sound travel time.
It is important to consider these additional factors when determining sound travel time in real-world scenarios. Variations in temperature and humidity can significantly impact the accuracy of calculations and measurements, especially over long distances.
Understanding the variables that affect sound travel time allows for more accurate predictions and measurements in various applications. Whether it is in telecommunications, architectural acoustics, or environmental monitoring, accounting for distance, temperature, and humidity ensures the reliability of sound-based measurements and calculations. By incorporating these factors into our understanding of sound travel time, we can make informed decisions and predictions regarding the behavior of sound waves in different environments.
Formula for Calculating Sound Travel Time
Introduction to the speed-distance-time formula.
In order to understand how long it takes sound to travel a specific distance, it is important to be familiar with the speed-distance-time formula. This formula is commonly used to calculate the time it takes for an object to travel a certain distance at a given speed. It can be expressed as:
Time = Distance / Speed
This formula allows us to determine how long it will take for sound to travel a particular distance, provided we know the speed of sound in the medium being analyzed.
Presentation of the specific formula for sound
The formula for calculating the time it takes sound to travel a given distance is derived from the speed-distance-time formula. Since speed is equal to distance divided by time, we can rearrange the formula to solve for time:
Applying this to sound, the formula becomes:
Time = Distance / Speed of Sound
By substituting the distance in miles and the speed of sound in miles per hour, we can calculate the time it takes for sound to travel through a specific medium.
For example, if we want to know how long it takes sound to travel 1 mile in air, and the speed of sound in air is approximately 767 miles per hour, we can plug in the values into the formula:
Time = 1 mile / 767 miles per hour
Calculating this, we find that sound takes approximately 0.0013 hours or 4.67 seconds to travel 1 mile in air.
It is important to note that this is a simplified calculation and does not take into account variables such as temperature, humidity, or atmospheric conditions, which can affect the speed of sound. These factors can introduce variations in the actual sound travel time. However, the formula provides a basic understanding of how to estimate sound travel time in a given medium.
In the next section, we will examine a case study on sound travel time through water and explore how it compares to sound travel time in air.
Sound Travel Time in Air
Calculation of sound travel time in one mile of air.
In this section, we will explore the calculation of sound travel time in one mile of air and discuss any variations that may occur due to atmospheric conditions.
Sound travels through air as a mechanical wave, with compressions and rarefactions propagating through the medium. The speed of sound in air is affected by several factors, including temperature, humidity, and air pressure. However, under standard atmospheric conditions (20°C temperature, 50% relative humidity, and 1 atmosphere pressure), the speed of sound in dry air is approximately 343 meters per second or 767 miles per hour.
To calculate the time it takes for sound to travel a distance, we can use the formula:
Therefore, to determine the sound travel time in one mile of air, we divide the distance (1 mile) by the speed of sound in air (767 miles per hour):
Converting the distance to meters and the speed to meters per second, we get:
Time = 1609 meters / 343 meters per second
Simplifying the equation, we find:
Time = 4.69 seconds (approximately)
Hence, it takes approximately 4.69 seconds for sound to travel one mile in standard atmospheric conditions.
It’s important to note that the sound travel time in air can vary based on atmospheric conditions. For example, at higher temperatures, sound speed increases, decreasing the travel time. Similarly, changes in air pressure and humidity can also affect the speed of sound, leading to variations in travel time. These variations should be taken into account in practical applications where precise timing is crucial, such as in acoustic measurements and sound engineering.
Understanding the sound travel time in air is essential in various fields, including telecommunications, meteorology, and environmental monitoring. Additionally, it provides valuable insights into the behavior of sound waves, helping us comprehend how sound propagates through different mediums and its impact on our daily lives.
In the next section, we will examine a case study on sound travel time through water and compare it to air, further expanding our understanding of how different media affect the speed of sound.
Case Study: Sound Travel Time through Water
Examination of how sound travels in water compared to air.
In this section, we will explore how sound travels in water compared to air and discuss the differences in sound travel time between the two mediums. Understanding sound travel time through water is essential for various fields such as underwater acoustics, marine biology, and oceanography.
Sound travels differently in water than in air due to the difference in the medium’s properties. In water, sound waves travel through a denser medium, which leads to higher speed and greater efficiency compared to air. Water molecules are closer to each other, allowing sound waves to propagate more efficiently.
Unlike air, water is a denser medium that allows sound waves to travel faster. The speed of sound in water is approximately 1,482 meters per second (4,855 feet per second), which is about four times faster than in air. This means that sound can travel through water over four times faster than it can through air.
Calculation of sound travel time in one mile of water
To calculate the sound travel time in one mile of water, we can use the formula: time = distance / speed. Since sound travels at approximately 1,482 meters per second in water, which is equivalent to 4,855 feet per second, we can calculate the time it takes for sound to travel one mile in water.
One mile is equivalent to 5,280 feet. By dividing this distance by the speed of sound in water (4,855 feet per second), we find that it takes about 1.09 seconds for sound to travel one mile through water.
It is important to note that this calculation represents the average speed of sound in water and may vary depending on factors such as temperature, salinity, and depth. Due to the different properties of water, sound travels faster in colder and saltier water compared to warmer and less salty water. Changes in depth can also affect the speed of sound in water.
Understanding sound travel time in water is crucial for various studies and applications. In underwater acoustics, for example, knowing the speed of sound in water helps in analyzing and interpreting underwater signals. Additionally, marine biologists and oceanographers use knowledge of sound travel time in water to study marine life and underwater geological features.
By examining the differences between sound travel in water and air, we can gain valuable insights into the unique properties of different mediums and their impact on the speed of sound. This knowledge allows us to better understand and navigate the underwater environment and utilize sound-based technologies effectively.
Sound Travel Time in Different Media
Comparison of sound travel times in various media.
Understanding how sound travels in different media is crucial in many fields, including physics, engineering, and communication. Sound waves travel at different speeds depending on the properties of the medium through which they pass. In this section, we will compare sound travel times in various media and discuss how different properties of these media affect the speed of sound.
One mile is commonly used as a standard unit to compare sound travel times across different media. In air, sound travels at a speed of approximately 343 meters per second (or 1,125 feet per second). However, sound travels faster in denser media, such as liquids and solids. In water, for example, sound travels at a speed of about 1,484 meters per second (or 4,872 feet per second), which is more than four times faster than in air.
Discussion on how media properties affect the speed of sound
The speed of sound in a medium is determined by two main properties: density and elasticity. Density refers to the amount of mass per unit volume, while elasticity refers to the medium’s ability to deform and regain its original shape. These properties directly affect how quickly sound waves can propagate through a medium.
In general, higher density slows down the speed of sound. Therefore, in media with higher densities, such as water and solids, sound waves travel faster compared to air, which has lower density. On the other hand, higher elasticity tends to increase the speed of sound. Solids, for example, have high elasticity, allowing sound waves to travel at higher speeds.
Besides density and elasticity, other factors can also influence the speed of sound in different media. Temperature, for instance, affects the speed of sound by altering the density and elasticity of the medium. Warmer temperatures generally result in faster sound speeds, while cooler temperatures lead to slower sound speeds.
In conclusion, understanding how sound travels in different media is essential for various applications. By comparing sound travel times and analyzing the properties of different media, scientists, engineers, and researchers can make accurate predictions and calculations. This knowledge finds practical applications in fields such as seismology, underwater acoustics, and music production. Overall, the speed of sound in various media is a fascinating topic that highlights the profound impact of material properties on the propagation of sound waves.
X. Real-life Applications
Explanation of practical uses of understanding sound travel time.
Understanding the concept of sound travel time has numerous real-life applications across various fields. By comprehending how long it takes for sound to travel a specific distance, scientists, engineers, and other professionals can make informed decisions and develop effective solutions in their respective domains.
One practical application of understanding sound travel time is in the field of underwater acoustics. Sound is used as a valuable tool for navigation, communication, and research in underwater environments. By knowing the speed of sound in water and understanding how it changes with temperature, salinity, and depth, scientists can accurately calculate the distance to underwater objects and determine their characteristics. This knowledge is crucial for mapping the ocean floor, studying marine life, and detecting underwater hazards.
Another field where knowledge of sound travel time is essential is seismology. Earthquakes generate seismic waves that travel through the Earth’s layers, providing valuable information about the Earth’s structure and the nature of the earthquake itself. By measuring the time it takes for seismic waves to reach different seismographs, scientists can accurately determine the location, magnitude, and depth of earthquakes. This information is crucial for earthquake early warning systems, assessing the potential damage of an earthquake, and understanding tectonic processes.
Examples in fields like underwater acoustics and seismology
In the field of underwater acoustics, understanding sound travel time is crucial for applications such as submarine navigation, underwater communication systems, and marine mammal research. For example, submarines rely on acoustic signals to navigate and communicate underwater. By accurately estimating the time it takes for sound to travel through water, submarines can calculate their distance from other underwater objects and avoid collisions.
In seismology, scientists utilize sound travel time to study earthquake waves and make predictions about future seismic events. Seismic waves travel at different speeds through different types of rocks, allowing seismologists to infer the geological properties of the Earth’s interior. By analyzing the travel times of seismic waves recorded by seismographs, scientists can create detailed maps of the Earth’s structure and identify potential earthquake zones.
Understanding sound travel time also has implications in industries such as architecture and transportation. In architecture, professionals consider the acoustics of a space to create optimal sound quality and minimize echoes and reverberation. By understanding how sound travels through different materials, architects can design spaces that enhance speech intelligibility and create pleasant listening environments.
In transportation, engineers need to consider the speed of sound when designing warning systems for level crossings, road work zones, and intersections. By accurately estimating the time it takes for sound to travel to a driver’s ears, engineers can ensure timely and effective warnings to prevent accidents.
In conclusion, understanding sound travel time has practical applications in various fields such as underwater acoustics, seismology, architecture, and transportation. By leveraging this knowledge, professionals can make informed decisions, improve safety measures, and develop innovative solutions in their respective industries.
Limitations of Sound as a Measurement Tool
Identification of factors reducing accuracy of sound measurements.
Sound is a widely used tool for measurement in various fields, including acoustics, engineering, and environmental assessments. It is a valuable method for determining distances, detecting objects, and analyzing the properties of different materials. However, it is important to understand the limitations and potential errors associated with using sound as a measurement tool.
One of the main factors that can reduce the accuracy of sound measurements is interference. Interference occurs when sound waves interact with each other, resulting in the creation of new waves that may distort or mask the original signal. This can make it difficult to measure the exact time it takes for sound to travel a certain distance. Interference is particularly pronounced in environments with multiple sound sources or reflective surfaces, such as crowded rooms or outdoor areas with many complex structures.
Another factor that can affect the accuracy of sound measurements is atmospheric conditions. Changes in temperature, humidity, and air pressure can all impact the speed of sound, causing variations in travel time. These variations can introduce errors into measurements, especially when precise timing is crucial. Additionally, wind and air currents can cause sound to deviate from its intended path, leading to inaccuracies in distance calculations.
The equipment used to measure sound can also introduce errors and limitations. The quality and calibrations of microphones, speakers, and other sound recording and playback devices can affect the accuracy of measurements. Inaccurate or poorly maintained equipment may introduce noise or distortion into the recorded sound, making it challenging to obtain reliable measurements.
Discussion on potential errors and challenges
In addition to the factors outlined above, there are several other potential errors and challenges when using sound as a measurement tool. The presence of background noise can interfere with measurements, making it difficult to isolate and analyze specific sound signals. This is particularly relevant in urban areas or industrial environments where there are high levels of ambient noise.
Furthermore, the physical properties of the medium through which sound travels can impact measurement accuracy. Sound waves can be absorbed, scattered, or reflected by different materials, introducing variations in travel time and amplitude. This can make it challenging to obtain precise measurements, especially in environments with a complex mix of materials and surfaces.
Another challenge is the potential for human error. Sound measurements often require careful positioning of equipment, precise timing, and accurate data recording. Any mistakes in these processes can introduce errors into the measurements. It is crucial for researchers and practitioners to undergo proper training and follow standardized measurement protocols to minimize the risk of human error.
Overall, while sound is a powerful tool for measurement, it is important to recognize its limitations and potential sources of error. Understanding and accounting for these limitations is critical to ensure accurate and reliable results in various applications, including environmental monitoring, industrial testing, and acoustic design. Ongoing advancements in technology and measurement techniques continue to address these challenges and improve the accuracy of sound measurements.
Implications for Music and Sound Production
Exploration of how sound travel time affects music production.
Understanding the concept of sound travel time is crucial in the field of music and sound production. The delay or latency caused by sound traveling through space has a significant impact on the quality and experience of music. By grasping the implications of sound travel time, musicians and audio engineers can make informed decisions to enhance the overall production.
When sound is produced, it travels through the air at a speed of approximately 1,125 feet per second. This means that even a small distance between the sound source and the listener can result in a noticeable delay. In a live performance setting, the sound from an instrument or vocalist reaches different parts of the audience at slightly different times, which can create a muddled or disjointed listening experience.
Mention of techniques used to manage phase and timing
To manage the phase and timing issues caused by sound travel time, professionals in the music industry employ various techniques. One commonly used method is to strategically position speakers or microphones to minimize the delay at different listening positions. By considering the physical distances and angles, engineers can ensure a more synchronized sound for the audience.
Digital audio workstations (DAWs) also offer tools to address phase and timing challenges caused by sound travel time. Audio plugins and effects enable producers to manipulate the timing of individual tracks, aligning them and compensating for any delays. Additionally, techniques such as time-based effects (e.g., delay and reverb) can be utilized creatively to enhance the overall depth and spatial characteristics of a musical composition.
Furthermore, sound travel time has implications for recording and mixing processes. In multi-microphone setups, phase cancellation can occur when two or more microphones pick up the same sound source with a slight time delay. This can lead to a loss of certain frequencies or a thinning of the overall sound. Audio engineers must carefully manage microphone placement and adjust time-alignment settings to achieve a cohesive and full-bodied sound.
In conclusion, understanding sound travel time is crucial in the realm of music and sound production. The delay caused by the time it takes for sound to travel through space can significantly impact the listening experience. Musicians, audio engineers, and producers must consider and manage these implications to ensure high-quality and synchronized sound reproduction. By implementing techniques such as strategic positioning of speakers, utilizing DAW tools, and adjusting microphone placement, professionals can effectively address phase and timing issues and enhance the overall production.
Recap of key points discussed
Throughout this article, we have explored various aspects of sound travel time and its significance. We began by defining sound as a mechanical wave and understanding the basics of compression and rarefaction. We then delved into the speed of sound in air, highlighting the factors that affect its velocity and exploring the formula for calculating it. Additionally, we compared the speed of sound in air, water, and solids, and discussed how density and elasticity play a role in determining sound speed.
Moving on, we examined the variables that influence sound travel time, with a focus on distance and mentioning other factors like temperature and humidity. We also introduced the speed-distance-time formula, specifically applying it to calculate sound travel time in one mile of air. Furthermore, we conducted a case study on sound travel time through water, comparing it to air and determining the sound travel time in one mile of water.
Then, we discussed sound travel time in different media, highlighting how media properties can affect the speed of sound. Real-life applications of understanding sound travel time were also explored, with examples in underwater acoustics and seismology. We acknowledged the limitations of sound as a measurement tool, identifying factors that can reduce its accuracy and discussing potential errors and challenges.
Final thoughts on the significance of sound travel time
Understanding sound travel time is crucial in various fields and industries. In music production, for instance, knowing the time it takes for sound to travel allows producers to manage phase and timing, ensuring a cohesive and high-quality audio experience. Similarly, in fields like underwater acoustics and seismology, precise knowledge of sound travel time is vital for interpreting data and making accurate assessments.
Moreover, sound travel time has implications beyond practical applications. It is a fundamental aspect of our perception of the world around us. Whether it’s the sound of a thunderstorm rolling in or the distant chirping of birds, the time it takes for sound to reach our ears adds depth and context to our auditory experiences.
In conclusion, understanding how long it takes for sound to travel a distance is essential for various scientific, technical, and artistic endeavors. By comprehending the factors that affect sound travel time and applying the appropriate formulas, we can harness the power of sound and unlock its limitless possibilities. So, the next time you hear a sound, take a moment to appreciate the fascinating journey it embarked on to reach your ears.
Leave a Comment Cancel reply
Save my name, email, and website in this browser for the next time I comment.
How Long Does It Take Sound to Travel 1 Mile?

How Long Does Sound Take to Travel 1 Mile?
Have you ever wondered how long it takes for sound to travel from one place to another? It may seem like a simple question, but the answer is actually quite complex. The speed of sound depends on a number of factors, including the medium through which it is traveling. In this article, we will explore the factors that affect the speed of sound and provide a detailed answer to the question of how long it takes for sound to travel 1 mile.
The Speed of Sound
The speed of sound is defined as the distance that a sound wave travels per unit of time. In other words, it is the rate at which a sound wave propagates through a medium. The speed of sound is typically measured in meters per second (m/s).
The speed of sound is not constant and can vary depending on the medium through which it is traveling. The speed of sound is fastest in solids, followed by liquids, and then gases. This is because sound waves travel more easily through dense materials than through less dense materials.
The speed of sound also depends on the temperature of the medium. Sound waves travel faster through warmer materials than through cooler materials. This is because the molecules in warmer materials vibrate more quickly, which allows sound waves to travel more easily.
How Long Does it Take for Sound to Travel 1 Mile?
Now that we know what affects the speed of sound, we can answer the question of how long it takes for sound to travel 1 mile.
The speed of sound in air is approximately 1,125 feet per second (343 meters per second). This means that it takes sound approximately 5 seconds to travel 1 mile.
However, the speed of sound can vary depending on the conditions of the air. For example, the speed of sound is slower in cold air than in warm air. It is also slower in humid air than in dry air.
The speed of sound is a complex phenomenon that is affected by a number of factors. In this article, we have explored the factors that affect the speed of sound and provided a detailed answer to the question of how long it takes for sound to travel 1 mile.
“`html
Sound is a vibration that travels through a medium, such as air or water. The speed of sound is the distance that a sound wave travels in one second. The speed of sound in air at sea level is 767 miles per hour (1,235 kilometers per hour).
The speed of sound is affected by temperature, humidity, and air pressure. The speed of sound decreases as the temperature decreases, and increases as the humidity and air pressure increase.
The speed of sound is also affected by the medium through which it travels. The speed of sound is faster in solids than in liquids, and faster in liquids than in gases. The speed of sound is also affected by the density of the medium.
The speed of sound in air at sea level is 767 miles per hour (1,235 kilometers per hour). This means that it takes sound about 5 seconds to travel 1 mile.
For example, the speed of sound is about 1% slower at 32 degrees Fahrenheit (0 degrees Celsius) than it is at 68 degrees Fahrenheit (20 degrees Celsius). The speed of sound is also about 1% faster at 100% humidity than it is at 0% humidity.
Factors Affecting the Speed of Sound
The speed of sound is affected by a number of factors, including:
- Temperature: The speed of sound decreases as the temperature decreases. This is because the molecules in a substance vibrate more slowly at lower temperatures.
- Humidity: The speed of sound increases as the humidity increases. This is because the water molecules in the air absorb some of the sound energy, which causes the sound waves to travel faster.
- Air pressure: The speed of sound increases as the air pressure increases. This is because the denser air molecules are more likely to collide with each other and transfer their energy to the sound waves.
- Medium: The speed of sound is faster in solids than in liquids, and faster in liquids than in gases. This is because the molecules in a solid are packed more tightly together than the molecules in a liquid or gas.
- Density: The speed of sound is also affected by the density of the medium. The denser the medium, the faster the sound waves will travel.
The speed of sound is a fundamental property of matter. It is affected by a number of factors, including temperature, humidity, air pressure, and the medium through which it travels. The speed of sound is important in a variety of applications, such as acoustics, sonar, and meteorology.
How Long Does Sound Take To Travel 1 Mile?
The speed of sound is the distance that sound travels per unit of time. It is measured in meters per second (m/s). The speed of sound in air is about 343 m/s at sea level and 20 C (68 F).
The speed of sound varies depending on the medium through which it is traveling. It is slower in solids than in liquids, and slower in liquids than in gases. The speed of sound in water is about 1,480 m/s, and the speed of sound in steel is about 5,100 m/s.
The time it takes for sound to travel 1 mile depends on the medium through which it is traveling. In air, sound will travel 1 mile in about 5 seconds. In water, sound will travel 1 mile in about 1.5 seconds. In steel, sound will travel 1 mile in about 0.2 seconds.
The speed of sound is a fundamental property of the universe. It is used in a variety of applications, including sonar, radar, echolocation, musical instruments, and ultrasound.
Applications of the Speed of Sound
The speed of sound is used in a variety of applications, including:
Sonar is a technology that uses sound waves to detect objects underwater. Sonar works by sending out a sound wave and then listening for the echo that is reflected back from the object. The time it takes for the echo to return tells the sonar operator how far away the object is. Sonar is used by ships and submarines to navigate and avoid obstacles, and by fishermen to find fish.
Radar is a technology that uses radio waves to detect objects. Radar works by sending out a radio wave and then listening for the echo that is reflected back from the object. The time it takes for the echo to return tells the radar operator how far away the object is. Radar is used by aircraft and ships to navigate and avoid obstacles, and by law enforcement to track criminals.
- Echolocation
Echolocation is a natural ability that some animals have to use sound waves to navigate and find food. Echolocation works by sending out a sound wave and then listening for the echo that is reflected back from objects in the environment. The time it takes for the echo to return tells the animal how far away the object is. Echolocation is used by bats, dolphins, and whales to navigate and find food.
- Musical instruments
The speed of sound is used in a variety of musical instruments. The length of a string on a musical instrument determines the pitch of the note that it produces. The longer the string, the lower the pitch. This is because the speed of sound is slower in longer strings. The speed of sound is also used to tune musical instruments.
Ultrasound is a type of sound wave that has a frequency that is too high for humans to hear. Ultrasound is used in a variety of medical applications, including imaging, surgery, and therapy. Ultrasound imaging uses sound waves to create images of internal organs and structures. Ultrasound surgery uses sound waves to break up kidney stones and other blockages. Ultrasound therapy uses sound waves to treat pain and inflammation.
How long does sound take to travel 1 mile?
Sound travels at a speed of about 1,125 feet per second, or 767 miles per hour. This means that it takes sound about 5 seconds to travel 1 mile.
What factors affect the speed of sound?
The speed of sound is affected by several factors, including:
- The temperature of the air: Sound travels faster in warm air than in cold air.
- The humidity of the air: Sound travels slower in humid air than in dry air.
- The presence of obstacles: Sound travels slower in the presence of obstacles, such as trees or buildings.
How can I measure the speed of sound?
There are several ways to measure the speed of sound. One common method is to use a spark gap generator to create a short burst of sound. The sound wave is then reflected off of a distant object, and the time it takes for the sound to travel to the object and back is measured.
What are some applications of the speed of sound?
The speed of sound has a variety of applications, including:
- Sonar: Sonar uses sound waves to detect objects underwater.
- Radar: Radar uses radio waves to detect objects in the air.
- Acoustic communication: Acoustic communication uses sound waves to transmit information.
What is the difference between the speed of sound and the speed of light?
The speed of sound is much slower than the speed of light. The speed of sound is about 1,125 feet per second, or 767 miles per hour. The speed of light is about 186,282 miles per second.
What is the coolest thing about the speed of sound?
One of the coolest things about the speed of sound is that it can be used to create a sonic boom. A sonic boom is a loud noise that is created when an object travels faster than the speed of sound. Sonic booms are often created by aircraft, and they can be heard from miles away.
In this article, we have discussed the speed of sound and how it is affected by different factors. We have also seen how the speed of sound can be used to measure the distance to objects, such as lightning strikes and the Earth’s interior.
The speed of sound is a fundamental property of the universe, and it has a wide range of applications in science and technology. By understanding the speed of sound, we can better understand the world around us.
Here are some key takeaways from this article:
- The speed of sound in air is approximately 767 miles per hour (1,235 kilometers per hour).
- The speed of sound is affected by temperature, humidity, and air pressure.
- The speed of sound can be used to measure the distance to objects, such as lightning strikes and the Earth’s interior.
- The speed of sound is a fundamental property of the universe, and it has a wide range of applications in science and technology.
Author Profile
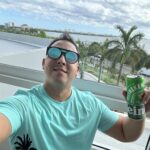
Latest entries
- January 19, 2024 Hiking How to Lace Hiking Boots for a Perfect Fit
- January 19, 2024 Camping How to Dispose of Camping Propane Tanks the Right Way
- January 19, 2024 Traveling Information Is Buffalo Still Under Travel Ban? (Updated for 2023)
- January 19, 2024 Cruise/Cruising Which Carnival Cruise Is Best for Families?
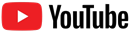
- TPC and eLearning
- Read Watch Interact
- What's NEW at TPC?
- Practice Review Test
- Teacher-Tools
- Subscription Selection
- Seat Calculator
- Ad Free Account
- Edit Profile Settings
- Classes (Version 2)
- Student Progress Edit
- Task Properties
- Export Student Progress
- Task, Activities, and Scores
- Metric Conversions Questions
- Metric System Questions
- Metric Estimation Questions
- Significant Digits Questions
- Proportional Reasoning
- Acceleration
- Distance-Displacement
- Dots and Graphs
- Graph That Motion
- Match That Graph
- Name That Motion
- Motion Diagrams
- Pos'n Time Graphs Numerical
- Pos'n Time Graphs Conceptual
- Up And Down - Questions
- Balanced vs. Unbalanced Forces
- Change of State
- Force and Motion
- Mass and Weight
- Match That Free-Body Diagram
- Net Force (and Acceleration) Ranking Tasks
- Newton's Second Law
- Normal Force Card Sort
- Recognizing Forces
- Air Resistance and Skydiving
- Solve It! with Newton's Second Law
- Which One Doesn't Belong?
- Component Addition Questions
- Head-to-Tail Vector Addition
- Projectile Mathematics
- Trajectory - Angle Launched Projectiles
- Trajectory - Horizontally Launched Projectiles
- Vector Addition
- Vector Direction
- Which One Doesn't Belong? Projectile Motion
- Forces in 2-Dimensions
- Being Impulsive About Momentum
- Explosions - Law Breakers
- Hit and Stick Collisions - Law Breakers
- Case Studies: Impulse and Force
- Impulse-Momentum Change Table
- Keeping Track of Momentum - Hit and Stick
- Keeping Track of Momentum - Hit and Bounce
- What's Up (and Down) with KE and PE?
- Energy Conservation Questions
- Energy Dissipation Questions
- Energy Ranking Tasks
- LOL Charts (a.k.a., Energy Bar Charts)
- Match That Bar Chart
- Words and Charts Questions
- Name That Energy
- Stepping Up with PE and KE Questions
- Case Studies - Circular Motion
- Circular Logic
- Forces and Free-Body Diagrams in Circular Motion
- Gravitational Field Strength
- Universal Gravitation
- Angular Position and Displacement
- Linear and Angular Velocity
- Angular Acceleration
- Rotational Inertia
- Balanced vs. Unbalanced Torques
- Getting a Handle on Torque
- Torque-ing About Rotation
- Properties of Matter
- Fluid Pressure
- Buoyant Force
- Sinking, Floating, and Hanging
- Pascal's Principle
- Flow Velocity
- Bernoulli's Principle
- Balloon Interactions
- Charge and Charging
- Charge Interactions
- Charging by Induction
- Conductors and Insulators
- Coulombs Law
- Electric Field
- Electric Field Intensity
- Polarization
- Case Studies: Electric Power
- Know Your Potential
- Light Bulb Anatomy
- I = ∆V/R Equations as a Guide to Thinking
- Parallel Circuits - ∆V = I•R Calculations
- Resistance Ranking Tasks
- Series Circuits - ∆V = I•R Calculations
- Series vs. Parallel Circuits
- Equivalent Resistance
- Period and Frequency of a Pendulum
- Pendulum Motion: Velocity and Force
- Energy of a Pendulum
- Period and Frequency of a Mass on a Spring
- Horizontal Springs: Velocity and Force
- Vertical Springs: Velocity and Force
- Energy of a Mass on a Spring
- Decibel Scale
- Frequency and Period
- Closed-End Air Columns
- Name That Harmonic: Strings
- Rocking the Boat
- Wave Basics
- Matching Pairs: Wave Characteristics
- Wave Interference
- Waves - Case Studies
- Color Addition and Subtraction
- Color Filters
- If This, Then That: Color Subtraction
- Light Intensity
- Color Pigments
- Converging Lenses
- Curved Mirror Images
- Law of Reflection
- Refraction and Lenses
- Total Internal Reflection
- Who Can See Who?
- Formulas and Atom Counting
- Atomic Models
- Bond Polarity
- Entropy Questions
- Cell Voltage Questions
- Heat of Formation Questions
- Reduction Potential Questions
- Oxidation States Questions
- Measuring the Quantity of Heat
- Hess's Law
- Oxidation-Reduction Questions
- Galvanic Cells Questions
- Thermal Stoichiometry
- Molecular Polarity
- Quantum Mechanics
- Balancing Chemical Equations
- Bronsted-Lowry Model of Acids and Bases
- Classification of Matter
- Collision Model of Reaction Rates
- Density Ranking Tasks
- Dissociation Reactions
- Complete Electron Configurations
- Elemental Measures
- Enthalpy Change Questions
- Equilibrium Concept
- Equilibrium Constant Expression
- Equilibrium Calculations - Questions
- Equilibrium ICE Table
- Intermolecular Forces Questions
- Ionic Bonding
- Lewis Electron Dot Structures
- Limiting Reactants
- Line Spectra Questions
- Mass Stoichiometry
- Measurement and Numbers
- Metals, Nonmetals, and Metalloids
- Metric Estimations
- Metric System
- Molarity Ranking Tasks
- Mole Conversions
- Name That Element
- Names to Formulas
- Names to Formulas 2
- Nuclear Decay
- Particles, Words, and Formulas
- Periodic Trends
- Precipitation Reactions and Net Ionic Equations
- Pressure Concepts
- Pressure-Temperature Gas Law
- Pressure-Volume Gas Law
- Chemical Reaction Types
- Significant Digits and Measurement
- States Of Matter Exercise
- Stoichiometry Law Breakers
- Stoichiometry - Math Relationships
- Subatomic Particles
- Spontaneity and Driving Forces
- Gibbs Free Energy
- Volume-Temperature Gas Law
- Acid-Base Properties
- Energy and Chemical Reactions
- Chemical and Physical Properties
- Valence Shell Electron Pair Repulsion Theory
- Writing Balanced Chemical Equations
- Mission CG1
- Mission CG10
- Mission CG2
- Mission CG3
- Mission CG4
- Mission CG5
- Mission CG6
- Mission CG7
- Mission CG8
- Mission CG9
- Mission EC1
- Mission EC10
- Mission EC11
- Mission EC12
- Mission EC2
- Mission EC3
- Mission EC4
- Mission EC5
- Mission EC6
- Mission EC7
- Mission EC8
- Mission EC9
- Mission RL1
- Mission RL2
- Mission RL3
- Mission RL4
- Mission RL5
- Mission RL6
- Mission KG7
- Mission RL8
- Mission KG9
- Mission RL10
- Mission RL11
- Mission RM1
- Mission RM2
- Mission RM3
- Mission RM4
- Mission RM5
- Mission RM6
- Mission RM8
- Mission RM10
- Mission LC1
- Mission RM11
- Mission LC2
- Mission LC3
- Mission LC4
- Mission LC5
- Mission LC6
- Mission LC8
- Mission SM1
- Mission SM2
- Mission SM3
- Mission SM4
- Mission SM5
- Mission SM6
- Mission SM8
- Mission SM10
- Mission KG10
- Mission SM11
- Mission KG2
- Mission KG3
- Mission KG4
- Mission KG5
- Mission KG6
- Mission KG8
- Mission KG11
- Mission F2D1
- Mission F2D2
- Mission F2D3
- Mission F2D4
- Mission F2D5
- Mission F2D6
- Mission KC1
- Mission KC2
- Mission KC3
- Mission KC4
- Mission KC5
- Mission KC6
- Mission KC7
- Mission KC8
- Mission AAA
- Mission SM9
- Mission LC7
- Mission LC9
- Mission NL1
- Mission NL2
- Mission NL3
- Mission NL4
- Mission NL5
- Mission NL6
- Mission NL7
- Mission NL8
- Mission NL9
- Mission NL10
- Mission NL11
- Mission NL12
- Mission MC1
- Mission MC10
- Mission MC2
- Mission MC3
- Mission MC4
- Mission MC5
- Mission MC6
- Mission MC7
- Mission MC8
- Mission MC9
- Mission RM7
- Mission RM9
- Mission RL7
- Mission RL9
- Mission SM7
- Mission SE1
- Mission SE10
- Mission SE11
- Mission SE12
- Mission SE2
- Mission SE3
- Mission SE4
- Mission SE5
- Mission SE6
- Mission SE7
- Mission SE8
- Mission SE9
- Mission VP1
- Mission VP10
- Mission VP2
- Mission VP3
- Mission VP4
- Mission VP5
- Mission VP6
- Mission VP7
- Mission VP8
- Mission VP9
- Mission WM1
- Mission WM2
- Mission WM3
- Mission WM4
- Mission WM5
- Mission WM6
- Mission WM7
- Mission WM8
- Mission WE1
- Mission WE10
- Mission WE2
- Mission WE3
- Mission WE4
- Mission WE5
- Mission WE6
- Mission WE7
- Mission WE8
- Mission WE9
- Vector Walk Interactive
- Name That Motion Interactive
- Kinematic Graphing 1 Concept Checker
- Kinematic Graphing 2 Concept Checker
- Graph That Motion Interactive
- Two Stage Rocket Interactive
- Rocket Sled Concept Checker
- Force Concept Checker
- Free-Body Diagrams Concept Checker
- Free-Body Diagrams The Sequel Concept Checker
- Skydiving Concept Checker
- Elevator Ride Concept Checker
- Vector Addition Concept Checker
- Vector Walk in Two Dimensions Interactive
- Name That Vector Interactive
- River Boat Simulator Concept Checker
- Projectile Simulator 2 Concept Checker
- Projectile Simulator 3 Concept Checker
- Hit the Target Interactive
- Turd the Target 1 Interactive
- Turd the Target 2 Interactive
- Balance It Interactive
- Go For The Gold Interactive
- Egg Drop Concept Checker
- Fish Catch Concept Checker
- Exploding Carts Concept Checker
- Collision Carts - Inelastic Collisions Concept Checker
- Its All Uphill Concept Checker
- Stopping Distance Concept Checker
- Chart That Motion Interactive
- Roller Coaster Model Concept Checker
- Uniform Circular Motion Concept Checker
- Horizontal Circle Simulation Concept Checker
- Vertical Circle Simulation Concept Checker
- Race Track Concept Checker
- Gravitational Fields Concept Checker
- Orbital Motion Concept Checker
- Angular Acceleration Concept Checker
- Balance Beam Concept Checker
- Torque Balancer Concept Checker
- Aluminum Can Polarization Concept Checker
- Charging Concept Checker
- Name That Charge Simulation
- Coulomb's Law Concept Checker
- Electric Field Lines Concept Checker
- Put the Charge in the Goal Concept Checker
- Circuit Builder Concept Checker (Series Circuits)
- Circuit Builder Concept Checker (Parallel Circuits)
- Circuit Builder Concept Checker (∆V-I-R)
- Circuit Builder Concept Checker (Voltage Drop)
- Equivalent Resistance Interactive
- Pendulum Motion Simulation Concept Checker
- Mass on a Spring Simulation Concept Checker
- Particle Wave Simulation Concept Checker
- Boundary Behavior Simulation Concept Checker
- Slinky Wave Simulator Concept Checker
- Simple Wave Simulator Concept Checker
- Wave Addition Simulation Concept Checker
- Standing Wave Maker Simulation Concept Checker
- Color Addition Concept Checker
- Painting With CMY Concept Checker
- Stage Lighting Concept Checker
- Filtering Away Concept Checker
- InterferencePatterns Concept Checker
- Young's Experiment Interactive
- Plane Mirror Images Interactive
- Who Can See Who Concept Checker
- Optics Bench (Mirrors) Concept Checker
- Name That Image (Mirrors) Interactive
- Refraction Concept Checker
- Total Internal Reflection Concept Checker
- Optics Bench (Lenses) Concept Checker
- Kinematics Preview
- Velocity Time Graphs Preview
- Moving Cart on an Inclined Plane Preview
- Stopping Distance Preview
- Cart, Bricks, and Bands Preview
- Fan Cart Study Preview
- Friction Preview
- Coffee Filter Lab Preview
- Friction, Speed, and Stopping Distance Preview
- Up and Down Preview
- Projectile Range Preview
- Ballistics Preview
- Juggling Preview
- Marshmallow Launcher Preview
- Air Bag Safety Preview
- Colliding Carts Preview
- Collisions Preview
- Engineering Safer Helmets Preview
- Push the Plow Preview
- Its All Uphill Preview
- Energy on an Incline Preview
- Modeling Roller Coasters Preview
- Hot Wheels Stopping Distance Preview
- Ball Bat Collision Preview
- Energy in Fields Preview
- Weightlessness Training Preview
- Roller Coaster Loops Preview
- Universal Gravitation Preview
- Keplers Laws Preview
- Kepler's Third Law Preview
- Charge Interactions Preview
- Sticky Tape Experiments Preview
- Wire Gauge Preview
- Voltage, Current, and Resistance Preview
- Light Bulb Resistance Preview
- Series and Parallel Circuits Preview
- Thermal Equilibrium Preview
- Linear Expansion Preview
- Heating Curves Preview
- Electricity and Magnetism - Part 1 Preview
- Electricity and Magnetism - Part 2 Preview
- Vibrating Mass on a Spring Preview
- Period of a Pendulum Preview
- Wave Speed Preview
- Slinky-Experiments Preview
- Standing Waves in a Rope Preview
- Sound as a Pressure Wave Preview
- DeciBel Scale Preview
- DeciBels, Phons, and Sones Preview
- Sound of Music Preview
- Shedding Light on Light Bulbs Preview
- Models of Light Preview
- Electromagnetic Radiation Preview
- Electromagnetic Spectrum Preview
- EM Wave Communication Preview
- Digitized Data Preview
- Light Intensity Preview
- Concave Mirrors Preview
- Object Image Relations Preview
- Snells Law Preview
- Reflection vs. Transmission Preview
- Magnification Lab Preview
- Reactivity Preview
- Ions and the Periodic Table Preview
- Periodic Trends Preview
- Intermolecular Forces Preview
- Melting Points and Boiling Points Preview
- Reaction Rates Preview
- Ammonia Factory Preview
- Stoichiometry Preview
- Nuclear Chemistry Preview
- Gaining Teacher Access
- Tasks and Classes
- Tasks - Classic
- Subscription
- Subscription Locator
- 1-D Kinematics
- Newton's Laws
- Vectors - Motion and Forces in Two Dimensions
- Momentum and Its Conservation
- Work and Energy
- Circular Motion and Satellite Motion
- Thermal Physics
- Static Electricity
- Electric Circuits
- Vibrations and Waves
- Sound Waves and Music
- Light and Color
- Reflection and Mirrors
- About the Physics Interactives
- Task Tracker
- Usage Policy
- Newtons Laws
- Vectors and Projectiles
- Forces in 2D
- Momentum and Collisions
- Circular and Satellite Motion
- Balance and Rotation
- Electromagnetism
- Waves and Sound
- Atomic Physics
- Forces in Two Dimensions
- Work, Energy, and Power
- Circular Motion and Gravitation
- Sound Waves
- 1-Dimensional Kinematics
- Circular, Satellite, and Rotational Motion
- Einstein's Theory of Special Relativity
- Waves, Sound and Light
- QuickTime Movies
- About the Concept Builders
- Pricing For Schools
- Directions for Version 2
- Measurement and Units
- Relationships and Graphs
- Rotation and Balance
- Vibrational Motion
- Reflection and Refraction
- Teacher Accounts
- Task Tracker Directions
- Kinematic Concepts
- Kinematic Graphing
- Wave Motion
- Sound and Music
- About CalcPad
- 1D Kinematics
- Vectors and Forces in 2D
- Simple Harmonic Motion
- Rotational Kinematics
- Rotation and Torque
- Rotational Dynamics
- Electric Fields, Potential, and Capacitance
- Transient RC Circuits
- Light Waves
- Units and Measurement
- Stoichiometry
- Molarity and Solutions
- Thermal Chemistry
- Acids and Bases
- Kinetics and Equilibrium
- Solution Equilibria
- Oxidation-Reduction
- Nuclear Chemistry
- NGSS Alignments
- 1D-Kinematics
- Projectiles
- Circular Motion
- Magnetism and Electromagnetism
- Graphing Practice
- About the ACT
- ACT Preparation
- For Teachers
- Other Resources
- Newton's Laws of Motion
- Work and Energy Packet
- Static Electricity Review
- Solutions Guide
- Solutions Guide Digital Download
- Motion in One Dimension
- Work, Energy and Power
- Frequently Asked Questions
- Purchasing the Download
- Purchasing the CD
- Purchasing the Digital Download
- About the NGSS Corner
- NGSS Search
- Force and Motion DCIs - High School
- Energy DCIs - High School
- Wave Applications DCIs - High School
- Force and Motion PEs - High School
- Energy PEs - High School
- Wave Applications PEs - High School
- Crosscutting Concepts
- The Practices
- Physics Topics
- NGSS Corner: Activity List
- NGSS Corner: Infographics
- About the Toolkits
- Position-Velocity-Acceleration
- Position-Time Graphs
- Velocity-Time Graphs
- Newton's First Law
- Newton's Second Law
- Newton's Third Law
- Terminal Velocity
- Projectile Motion
- Forces in 2 Dimensions
- Impulse and Momentum Change
- Momentum Conservation
- Work-Energy Fundamentals
- Work-Energy Relationship
- Roller Coaster Physics
- Satellite Motion
- Electric Fields
- Circuit Concepts
- Series Circuits
- Parallel Circuits
- Describing-Waves
- Wave Behavior Toolkit
- Standing Wave Patterns
- Resonating Air Columns
- Wave Model of Light
- Plane Mirrors
- Curved Mirrors
- Teacher Guide
- Using Lab Notebooks
- Current Electricity
- Light Waves and Color
- Reflection and Ray Model of Light
- Refraction and Ray Model of Light
- Classes (Legacy Version)
- Teacher Resources
- Subscriptions

- Newton's Laws
- Einstein's Theory of Special Relativity
- About Concept Checkers
- School Pricing
- Newton's Laws of Motion
- Newton's First Law
- Newton's Third Law
- The Speed of Sound
- Pitch and Frequency
- Intensity and the Decibel Scale
- The Human Ear
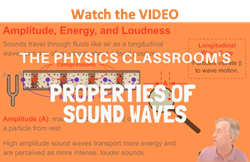
Since the speed of a wave is defined as the distance that a point on a wave (such as a compression or a rarefaction) travels per unit of time, it is often expressed in units of meters/second (abbreviated m/s). In equation form, this is
The faster a sound wave travels, the more distance it will cover in the same period of time. If a sound wave were observed to travel a distance of 700 meters in 2 seconds, then the speed of the wave would be 350 m/s. A slower wave would cover less distance - perhaps 660 meters - in the same time period of 2 seconds and thus have a speed of 330 m/s. Faster waves cover more distance in the same period of time.
Factors Affecting Wave Speed
The speed of any wave depends upon the properties of the medium through which the wave is traveling. Typically there are two essential types of properties that affect wave speed - inertial properties and elastic properties. Elastic properties are those properties related to the tendency of a material to maintain its shape and not deform whenever a force or stress is applied to it. A material such as steel will experience a very small deformation of shape (and dimension) when a stress is applied to it. Steel is a rigid material with a high elasticity. On the other hand, a material such as a rubber band is highly flexible; when a force is applied to stretch the rubber band, it deforms or changes its shape readily. A small stress on the rubber band causes a large deformation. Steel is considered to be a stiff or rigid material, whereas a rubber band is considered a flexible material. At the particle level, a stiff or rigid material is characterized by atoms and/or molecules with strong attractions for each other. When a force is applied in an attempt to stretch or deform the material, its strong particle interactions prevent this deformation and help the material maintain its shape. Rigid materials such as steel are considered to have a high elasticity. (Elastic modulus is the technical term). The phase of matter has a tremendous impact upon the elastic properties of the medium. In general, solids have the strongest interactions between particles, followed by liquids and then gases. For this reason, longitudinal sound waves travel faster in solids than they do in liquids than they do in gases. Even though the inertial factor may favor gases, the elastic factor has a greater influence on the speed ( v ) of a wave, thus yielding this general pattern:
Inertial properties are those properties related to the material's tendency to be sluggish to changes in its state of motion. The density of a medium is an example of an inertial property . The greater the inertia (i.e., mass density) of individual particles of the medium, the less responsive they will be to the interactions between neighboring particles and the slower that the wave will be. As stated above, sound waves travel faster in solids than they do in liquids than they do in gases. However, within a single phase of matter, the inertial property of density tends to be the property that has a greatest impact upon the speed of sound. A sound wave will travel faster in a less dense material than a more dense material. Thus, a sound wave will travel nearly three times faster in Helium than it will in air. This is mostly due to the lower mass of Helium particles as compared to air particles.
The Speed of Sound in Air
The speed of a sound wave in air depends upon the properties of the air, mostly the temperature, and to a lesser degree, the humidity. Humidity is the result of water vapor being present in air. Like any liquid, water has a tendency to evaporate. As it does, particles of gaseous water become mixed in the air. This additional matter will affect the mass density of the air (an inertial property). The temperature will affect the strength of the particle interactions (an elastic property). At normal atmospheric pressure, the temperature dependence of the speed of a sound wave through dry air is approximated by the following equation:
where T is the temperature of the air in degrees Celsius. Using this equation to determine the speed of a sound wave in air at a temperature of 20 degrees Celsius yields the following solution.
v = 331 m/s + (0.6 m/s/C)•(20 C)
v = 331 m/s + 12 m/s
v = 343 m/s
(The above equation relating the speed of a sound wave in air to the temperature provides reasonably accurate speed values for temperatures between 0 and 100 Celsius. The equation itself does not have any theoretical basis; it is simply the result of inspecting temperature-speed data for this temperature range. Other equations do exist that are based upon theoretical reasoning and provide accurate data for all temperatures. Nonetheless, the equation above will be sufficient for our use as introductory Physics students.)
Look It Up!
Using wave speed to determine distances.
At normal atmospheric pressure and a temperature of 20 degrees Celsius, a sound wave will travel at approximately 343 m/s; this is approximately equal to 750 miles/hour. While this speed may seem fast by human standards (the fastest humans can sprint at approximately 11 m/s and highway speeds are approximately 30 m/s), the speed of a sound wave is slow in comparison to the speed of a light wave. Light travels through air at a speed of approximately 300 000 000 m/s; this is nearly 900 000 times the speed of sound. For this reason, humans can observe a detectable time delay between the thunder and the lightning during a storm. The arrival of the light wave from the location of the lightning strike occurs in so little time that it is essentially negligible. Yet the arrival of the sound wave from the location of the lightning strike occurs much later. The time delay between the arrival of the light wave (lightning) and the arrival of the sound wave (thunder) allows a person to approximate his/her distance from the storm location. For instance if the thunder is heard 3 seconds after the lightning is seen, then sound (whose speed is approximated as 345 m/s) has traveled a distance of
If this value is converted to miles (divide by 1600 m/1 mi), then the storm is a distance of 0.65 miles away.
Another phenomenon related to the perception of time delays between two events is an echo . A person can often perceive a time delay between the production of a sound and the arrival of a reflection of that sound off a distant barrier. If you have ever made a holler within a canyon, perhaps you have heard an echo of your holler off a distant canyon wall. The time delay between the holler and the echo corresponds to the time for the holler to travel the round-trip distance to the canyon wall and back. A measurement of this time would allow a person to estimate the one-way distance to the canyon wall. For instance if an echo is heard 1.40 seconds after making the holler , then the distance to the canyon wall can be found as follows:
The canyon wall is 242 meters away. You might have noticed that the time of 0.70 seconds is used in the equation. Since the time delay corresponds to the time for the holler to travel the round-trip distance to the canyon wall and back, the one-way distance to the canyon wall corresponds to one-half the time delay.
While an echo is of relatively minimal importance to humans, echolocation is an essential trick of the trade for bats. Being a nocturnal creature, bats must use sound waves to navigate and hunt. They produce short bursts of ultrasonic sound waves that reflect off objects in their surroundings and return. Their detection of the time delay between the sending and receiving of the pulses allows a bat to approximate the distance to surrounding objects. Some bats, known as Doppler bats, are capable of detecting the speed and direction of any moving objects by monitoring the changes in frequency of the reflected pulses. These bats are utilizing the physics of the Doppler effect discussed in an earlier unit (and also to be discussed later in Lesson 3 ). This method of echolocation enables a bat to navigate and to hunt.
The Wave Equation Revisited
Like any wave, a sound wave has a speed that is mathematically related to the frequency and the wavelength of the wave. As discussed in a previous unit , the mathematical relationship between speed, frequency and wavelength is given by the following equation.
Using the symbols v , λ , and f , the equation can be rewritten as
Check Your Understanding
1. An automatic focus camera is able to focus on objects by use of an ultrasonic sound wave. The camera sends out sound waves that reflect off distant objects and return to the camera. A sensor detects the time it takes for the waves to return and then determines the distance an object is from the camera. If a sound wave (speed = 340 m/s) returns to the camera 0.150 seconds after leaving the camera, how far away is the object?
Answer = 25.5 m
The speed of the sound wave is 340 m/s. The distance can be found using d = v • t resulting in an answer of 25.5 m. Use 0.075 seconds for the time since 0.150 seconds refers to the round-trip distance.
2. On a hot summer day, a pesky little mosquito produced its warning sound near your ear. The sound is produced by the beating of its wings at a rate of about 600 wing beats per second.
a. What is the frequency in Hertz of the sound wave? b. Assuming the sound wave moves with a velocity of 350 m/s, what is the wavelength of the wave?
Part a Answer: 600 Hz (given)
Part b Answer: 0.583 meters
3. Doubling the frequency of a wave source doubles the speed of the waves.
a. True b. False
Doubling the frequency will halve the wavelength; speed is unaffected by the alteration in the frequency. The speed of a wave depends upon the properties of the medium.
4. Playing middle C on the piano keyboard produces a sound with a frequency of 256 Hz. Assuming the speed of sound in air is 345 m/s, determine the wavelength of the sound corresponding to the note of middle C.
Answer: 1.35 meters (rounded)
Let λ = wavelength. Use v = f • λ where v = 345 m/s and f = 256 Hz. Rearrange the equation to the form of λ = v / f. Substitute and solve.
5. Most people can detect frequencies as high as 20 000 Hz. Assuming the speed of sound in air is 345 m/s, determine the wavelength of the sound corresponding to this upper range of audible hearing.
Answer: 0.0173 meters (rounded)
Let λ = wavelength. Use v = f • λ where v = 345 m/s and f = 20 000 Hz. Rearrange the equation to the form of λ = v / f. Substitute and solve.
6. An elephant produces a 10 Hz sound wave. Assuming the speed of sound in air is 345 m/s, determine the wavelength of this infrasonic sound wave.
Answer: 34.5 meters
Let λ = wavelength. Use v = f • λ where v = 345 m/s and f = 10 Hz. Rearrange the equation to the form of λ = v / f. Substitute and solve.
7. Determine the speed of sound on a cold winter day (T=3 degrees C).
Answer: 332.8 m/s
The speed of sound in air is dependent upon the temperature of air. The dependence is expressed by the equation:
v = 331 m/s + (0.6 m/s/C) • T
where T is the temperature in Celsius. Substitute and solve.
v = 331 m/s + (0.6 m/s/C) • 3 C v = 331 m/s + 1.8 m/s v = 332.8 m/s
8. Miles Tugo is camping in Glacier National Park. In the midst of a glacier canyon, he makes a loud holler. He hears an echo 1.22 seconds later. The air temperature is 20 degrees C. How far away are the canyon walls?
Answer = 209 m
The speed of the sound wave at this temperature is 343 m/s (using the equation described in the Tutorial). The distance can be found using d = v • t resulting in an answer of 343 m. Use 0.61 second for the time since 1.22 seconds refers to the round-trip distance.
9. Two sound waves are traveling through a container of unknown gas. Wave A has a wavelength of 1.2 m. Wave B has a wavelength of 3.6 m. The velocity of wave B must be __________ the velocity of wave A.
a. one-ninth b. one-third c. the same as d. three times larger than
The speed of a wave does not depend upon its wavelength, but rather upon the properties of the medium. The medium has not changed, so neither has the speed.
10. Two sound waves are traveling through a container of unknown gas. Wave A has a wavelength of 1.2 m. Wave B has a wavelength of 3.6 m. The frequency of wave B must be __________ the frequency of wave A.
Since Wave B has three times the wavelength of Wave A, it must have one-third the frequency. Frequency and wavelength are inversely related.
- Interference and Beats

How Far Does Sound Travel: The Science of Acoustics
Do you ever stop to think about how sound travels? It’s an interesting phenomenon that occurs everyday and yet we often take it for granted. In this blog post, we will explore the science of acoustics and how sound travels. We will answer the question of how far sound can travel and how it is affected by different factors. Stay tuned for an in-depth look at this fascinating topic!

Nature Of Sound
Sound is a mechanical wave that is an oscillation of pressure transmitted through some medium, such as air or water. Sound can propagate through solids and liquids better than gases because the density and stiffness are greater. So how far does sound travel? In this article we will answer how sound travels and how to calculate how far it travels in different scenarios.
Sound Transmits Conception
A common misconception with regard to how sound transmits itself between two points (for example from speaker to ear) is that the source creates waves of compression in the surrounding gas which then proceed on their way at a constant speed until they strike something else; either another solid object or our ears . This analogy might be okay for describing what goes on at low frequencies but once we go beyond around 1000 Hz, the propagation of sound becomes far more complex.

At low frequencies (below around 1000 Hz), sound waves tend to travel in all directions more or less equally and bounce off objects like a rubber ball would. As frequency increases however, the directivity of sound increases as well. So high-frequency sounds are more likely to travel in a straight line between two points than low frequencies. This is why we can often hear someone calling from some distance away when there is loud music playing – because the higher frequencies carry further than the lower ones.
How Far Can Sound Travel
There are three ways that sound can be transmitted: through air, through water, or through solids. The speed of sound through each medium is different and depends on the density and stiffness of the material.

The speed of sound through air is about 343 m/s (or 760 mph), and it travels faster in warmer air than colder air. The speed of sound through water is about 1500 m/s, and it travels faster in salt water than fresh water. The speed of sound through solids is much faster than through either gases or liquids – about 5000-15000 m/s. This is why we can often hear someone coming before we see them – the sound waves are travelling through the solid ground to our ears!
Now that we know how sound propagates and how its speed depends on the medium, let’s take a look at how to calculate how far it will travel between two points. We can use the equation
distance = speed x time
For example, if we want to know how far a sound will travel in one second, we have:
distance = 343 m/s x 0.001 s = 343 m
So sound travels 1 kilometer in roughly 3 seconds and 1 mile in roughly 5 seconds.
Does Вecibel Level affect the Sound Distance?
The surface area around a sound source’s location grows with the square of the distance from the source. This implies that the same amount of sound energy is dispersed over a larger surface, and that the energy intensity decreases as the square of the distance from the source (Inverse Square Law).
Experts of Acoustical control says, that
For every doubling of distance, the sound level reduces by 6 decibels (dB), (e.g. moving from 10 to 20 metres away from a sound source). But the next 6dB reduction means moving from 20 to 40 metres, then from 40 to 80 metres for a further 6dB reduction.
How Far Can Sound Travel In Real World
In real world, there are many factors that can affect how far a sound travels. Factors such as air density, temperature and humidity have an impact on its propagation; obstacles like buildings or mountains could also block some frequencies from going through while letting others pass (this happens because at high frequencies they behave more like waves).
Sounds can propagate through solids better than they can propagate through air because their density/stiffness are greater (this means that sound travels faster). In addition to this, we also know that it takes less time for a high frequency wave to reach us from its source compared with low frequencies. For example if there’s some kind of obstacle blocking our path then it might take longer for waves at higher frequencies than those below 1000 Hz to past them.
Can Sound Waves Travel Infinitely?
No. The higher the frequency of a sound wave, the shorter its wavelength becomes. As wavelength decreases, the amount of energy in a sound wave also decreases and eventually it dissipates completely. This is why we often can’t hear someone calling from very far away when there’s loud music playing – because the high frequencies are being blocked out by all the noise!
Can Sound Travel 20 Miles?
The air may be permeable to these lower-frequency, sub-audible sound waves generated by elephants. Some whale species’ frequencies might travel through seawater for 1500 kilometers or 900 miles.
How Far Can a Human Scream Travel?
The normal intelligible outdoor range of the male human voice in still air is 180 m (590 ft 6.6 in).

The Guiness World Record of the Farthest distance travelled by a human voice belongs the Spanish-speaking inhabitants of the Canary Island of La Gomera, is intelligible under ideal conditions at 8 km (5 miles).
In Conclusion
At the end of this blog post, you should have a better understanding of how sound travel and what factors affect it. If you want to learn more about acoustics and sounds, you can check out our resources here.
- Why PS4 Is So Loud and How to Fix It
- Best Quiet Electric Toothbrush – Buyer’s Guide
Ocean Noise Pollution: What Is It?
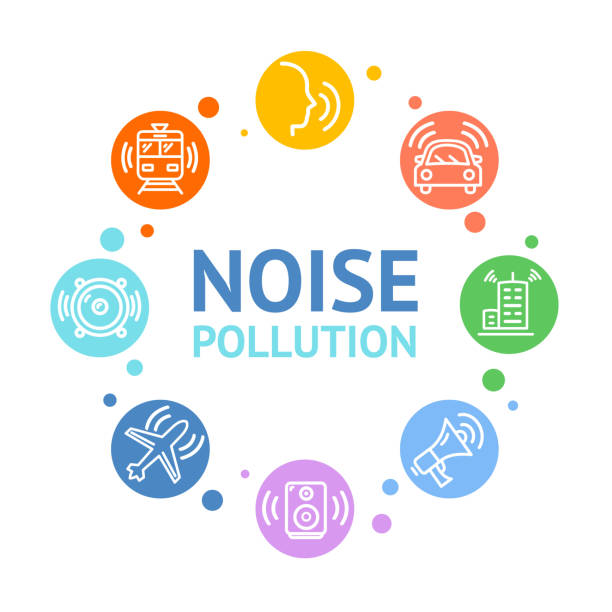
Ways to Reduce Noise Pollution in Your Life

Top 8 Organizations Against Noise Pollution
Lightning Distance Calculator
Table of contents
Our lightning distance calculator will help you estimate storm distances .
Imagine a cloudy, sultry summer afternoon. You see that the storm is approaching – suddenly, there's a dazzling lightning strike and, a few moments later, a deafening thunderclap. Are you in a safe place? How far away is the lightning? We may easily calculate the distance from the time difference between noticing the lightning flash and thunder.
If you are still unsure how to calculate lightning distance, keep reading and try the calculator!
How to calculate the lightning distance?
Light travels at 186,291 miles per second (~300,000 km/s), and the speed of sound in dry air is equal to 0.21-0.22 miles per second (0.33-0.35 km/s), as it varies with the temperature.
We can notice the lightning almost immediately even though the storm is far from us, but the thunder needs around 5 seconds to travel one mile and approximately 3 seconds to travel one kilometer. Thanks to this seven orders of magnitude difference in sound and light propagation, we can easily estimate the storm distance:
Storm distance = Time × Speed of sound ,
where Time is the number of seconds between noticing the flash and the thunder, and Speed of sound is the speed of sound in the air at a specific temperature. If you know how warm it was during that stormy day, you can check the speed value in our speed of sound calculator and use that exact number.
Did you notice that it's nothing else than a transformed simple formula for speed? For the more general formula for speed, view the speed calculator .
Storm distance – how far away is lightning? ⛈️
The method of counting the seconds between lightning and thunder is sometimes called the flash-to-bang technique. So how far is the lightning?
Watch the stormy sky . Hopefully, you are at some distance from the thunderstorm, not in the middle!
As soon as you notice the lightning strike, count the number of seconds until you hear the thunder . Use your watch or phone, or try to count seconds as accurately as possible. Assume that, in our case, it's 15 seconds.
Calculate the storm distance . If you want to know approximately how far away the lightning is in miles, divide the number of seconds by 5; for kilometers, divide the seconds by 3. It will give us 3 miles or 5 kilometers.
Or simply use our lightning distance calculator , which can calculate it more accurately at the speed of light !
If you are keen on atmospheric studies, have a look at our dew point calculator .
How do I calculate the distance of lightning in km?
To calculate the lightning distance in kilometers:
- Note the time in seconds between the lightning flash and the thunderclap .
- Divide the number of seconds by 3 .
- That's it! What you've found is the lightning distance in km.
How do I calculate the distance of lightning in miles?
To calculate the lightning distance in miles:
- Measure the time in seconds between the lightning flash and the thunderclap .
- Divide the number of seconds by 5 .
- Well done. You've just measured the lightning distance in miles.
What is the 30-30 rule for lightning?
When you see lightning, start counting to 30 . If you hear the thunder before you finish counting, the thunderstorm is close enough to be dangerous, so seek shelter . Go outside only after 30 minutes from the last thunderclap.
How long do I have to wait between thunder?
You must wait for at least 30 minutes after the last thunderclap as there may still be electric charges lingering in the clouds. This means there is still a chance of another lightning strike.
How fast is a lightning bolt?
Lightning bolts travel at the speed of light, which is 186,291 miles per second (~300,000 km/s) .
Can lightning heat up the surrounding air?
Lightning can heat the air surrounding it to over five times the temperature of the sun's surface (around 30,000 °C ).
Time between flash and thunder
Time difference in seconds.
Storm distance
.css-slt4t3.css-slt4t3{color:#2B3148;background-color:transparent;font-family:"Roboto","Helvetica","Arial",sans-serif;font-size:20px;line-height:24px;overflow:visible;padding-top:0px;position:relative;}.css-slt4t3.css-slt4t3:after{content:'';-webkit-transform:scale(0);-moz-transform:scale(0);-ms-transform:scale(0);transform:scale(0);position:absolute;border:2px solid #EA9430;border-radius:2px;inset:-8px;z-index:1;}.css-slt4t3 .js-external-link-button.link-like,.css-slt4t3 .js-external-link-anchor{color:inherit;border-radius:1px;-webkit-text-decoration:underline;text-decoration:underline;}.css-slt4t3 .js-external-link-button.link-like:hover,.css-slt4t3 .js-external-link-anchor:hover,.css-slt4t3 .js-external-link-button.link-like:active,.css-slt4t3 .js-external-link-anchor:active{text-decoration-thickness:2px;text-shadow:1px 0 0;}.css-slt4t3 .js-external-link-button.link-like:focus-visible,.css-slt4t3 .js-external-link-anchor:focus-visible{outline:transparent 2px dotted;box-shadow:0 0 0 2px #6314E6;}.css-slt4t3 p,.css-slt4t3 div{margin:0px;display:block;}.css-slt4t3 pre{margin:0px;display:block;}.css-slt4t3 pre code{display:block;width:-webkit-fit-content;width:-moz-fit-content;width:fit-content;}.css-slt4t3 pre:not(:first-child){padding-top:8px;}.css-slt4t3 ul,.css-slt4t3 ol{display:block margin:0px;padding-left:20px;}.css-slt4t3 ul li,.css-slt4t3 ol li{padding-top:8px;}.css-slt4t3 ul ul,.css-slt4t3 ol ul,.css-slt4t3 ul ol,.css-slt4t3 ol ol{padding-top:0px;}.css-slt4t3 ul:not(:first-child),.css-slt4t3 ol:not(:first-child){padding-top:4px;} .css-4okk7a{margin:auto;background-color:white;overflow:auto;overflow-wrap:break-word;word-break:break-word;}.css-4okk7a code,.css-4okk7a kbd,.css-4okk7a pre,.css-4okk7a samp{font-family:monospace;}.css-4okk7a code{padding:2px 4px;color:#444;background:#ddd;border-radius:4px;}.css-4okk7a figcaption,.css-4okk7a caption{text-align:center;}.css-4okk7a figcaption{font-size:12px;font-style:italic;overflow:hidden;}.css-4okk7a h3{font-size:1.75rem;}.css-4okk7a h4{font-size:1.5rem;}.css-4okk7a .mathBlock{font-size:24px;-webkit-padding-start:4px;padding-inline-start:4px;}.css-4okk7a .mathBlock .katex{font-size:24px;text-align:left;}.css-4okk7a .math-inline{background-color:#f0f0f0;display:inline-block;font-size:inherit;padding:0 3px;}.css-4okk7a .videoBlock,.css-4okk7a .imageBlock{margin-bottom:16px;}.css-4okk7a .imageBlock__image-align--left,.css-4okk7a .videoBlock__video-align--left{float:left;}.css-4okk7a .imageBlock__image-align--right,.css-4okk7a .videoBlock__video-align--right{float:right;}.css-4okk7a .imageBlock__image-align--center,.css-4okk7a .videoBlock__video-align--center{display:block;margin-left:auto;margin-right:auto;clear:both;}.css-4okk7a .imageBlock__image-align--none,.css-4okk7a .videoBlock__video-align--none{clear:both;margin-left:0;margin-right:0;}.css-4okk7a .videoBlock__video--wrapper{position:relative;padding-bottom:56.25%;height:0;}.css-4okk7a .videoBlock__video--wrapper iframe{position:absolute;top:0;left:0;width:100%;height:100%;}.css-4okk7a .videoBlock__caption{text-align:left;}@font-face{font-family:'KaTeX_AMS';src:url(/katex-fonts/KaTeX_AMS-Regular.woff2) format('woff2'),url(/katex-fonts/KaTeX_AMS-Regular.woff) format('woff'),url(/katex-fonts/KaTeX_AMS-Regular.ttf) format('truetype');font-weight:normal;font-style:normal;}@font-face{font-family:'KaTeX_Caligraphic';src:url(/katex-fonts/KaTeX_Caligraphic-Bold.woff2) format('woff2'),url(/katex-fonts/KaTeX_Caligraphic-Bold.woff) format('woff'),url(/katex-fonts/KaTeX_Caligraphic-Bold.ttf) format('truetype');font-weight:bold;font-style:normal;}@font-face{font-family:'KaTeX_Caligraphic';src:url(/katex-fonts/KaTeX_Caligraphic-Regular.woff2) format('woff2'),url(/katex-fonts/KaTeX_Caligraphic-Regular.woff) format('woff'),url(/katex-fonts/KaTeX_Caligraphic-Regular.ttf) format('truetype');font-weight:normal;font-style:normal;}@font-face{font-family:'KaTeX_Fraktur';src:url(/katex-fonts/KaTeX_Fraktur-Bold.woff2) format('woff2'),url(/katex-fonts/KaTeX_Fraktur-Bold.woff) format('woff'),url(/katex-fonts/KaTeX_Fraktur-Bold.ttf) format('truetype');font-weight:bold;font-style:normal;}@font-face{font-family:'KaTeX_Fraktur';src:url(/katex-fonts/KaTeX_Fraktur-Regular.woff2) format('woff2'),url(/katex-fonts/KaTeX_Fraktur-Regular.woff) format('woff'),url(/katex-fonts/KaTeX_Fraktur-Regular.ttf) format('truetype');font-weight:normal;font-style:normal;}@font-face{font-family:'KaTeX_Main';src:url(/katex-fonts/KaTeX_Main-Bold.woff2) format('woff2'),url(/katex-fonts/KaTeX_Main-Bold.woff) format('woff'),url(/katex-fonts/KaTeX_Main-Bold.ttf) format('truetype');font-weight:bold;font-style:normal;}@font-face{font-family:'KaTeX_Main';src:url(/katex-fonts/KaTeX_Main-BoldItalic.woff2) format('woff2'),url(/katex-fonts/KaTeX_Main-BoldItalic.woff) format('woff'),url(/katex-fonts/KaTeX_Main-BoldItalic.ttf) format('truetype');font-weight:bold;font-style:italic;}@font-face{font-family:'KaTeX_Main';src:url(/katex-fonts/KaTeX_Main-Italic.woff2) format('woff2'),url(/katex-fonts/KaTeX_Main-Italic.woff) format('woff'),url(/katex-fonts/KaTeX_Main-Italic.ttf) format('truetype');font-weight:normal;font-style:italic;}@font-face{font-family:'KaTeX_Main';src:url(/katex-fonts/KaTeX_Main-Regular.woff2) format('woff2'),url(/katex-fonts/KaTeX_Main-Regular.woff) format('woff'),url(/katex-fonts/KaTeX_Main-Regular.ttf) format('truetype');font-weight:normal;font-style:normal;}@font-face{font-family:'KaTeX_Math';src:url(/katex-fonts/KaTeX_Math-BoldItalic.woff2) format('woff2'),url(/katex-fonts/KaTeX_Math-BoldItalic.woff) format('woff'),url(/katex-fonts/KaTeX_Math-BoldItalic.ttf) format('truetype');font-weight:bold;font-style:italic;}@font-face{font-family:'KaTeX_Math';src:url(/katex-fonts/KaTeX_Math-Italic.woff2) format('woff2'),url(/katex-fonts/KaTeX_Math-Italic.woff) format('woff'),url(/katex-fonts/KaTeX_Math-Italic.ttf) format('truetype');font-weight:normal;font-style:italic;}@font-face{font-family:'KaTeX_SansSerif';src:url(/katex-fonts/KaTeX_SansSerif-Bold.woff2) format('woff2'),url(/katex-fonts/KaTeX_SansSerif-Bold.woff) format('woff'),url(/katex-fonts/KaTeX_SansSerif-Bold.ttf) format('truetype');font-weight:bold;font-style:normal;}@font-face{font-family:'KaTeX_SansSerif';src:url(/katex-fonts/KaTeX_SansSerif-Italic.woff2) format('woff2'),url(/katex-fonts/KaTeX_SansSerif-Italic.woff) format('woff'),url(/katex-fonts/KaTeX_SansSerif-Italic.ttf) format('truetype');font-weight:normal;font-style:italic;}@font-face{font-family:'KaTeX_SansSerif';src:url(/katex-fonts/KaTeX_SansSerif-Regular.woff2) format('woff2'),url(/katex-fonts/KaTeX_SansSerif-Regular.woff) format('woff'),url(/katex-fonts/KaTeX_SansSerif-Regular.ttf) format('truetype');font-weight:normal;font-style:normal;}@font-face{font-family:'KaTeX_Script';src:url(/katex-fonts/KaTeX_Script-Regular.woff2) format('woff2'),url(/katex-fonts/KaTeX_Script-Regular.woff) format('woff'),url(/katex-fonts/KaTeX_Script-Regular.ttf) format('truetype');font-weight:normal;font-style:normal;}@font-face{font-family:'KaTeX_Size1';src:url(/katex-fonts/KaTeX_Size1-Regular.woff2) format('woff2'),url(/katex-fonts/KaTeX_Size1-Regular.woff) format('woff'),url(/katex-fonts/KaTeX_Size1-Regular.ttf) format('truetype');font-weight:normal;font-style:normal;}@font-face{font-family:'KaTeX_Size2';src:url(/katex-fonts/KaTeX_Size2-Regular.woff2) format('woff2'),url(/katex-fonts/KaTeX_Size2-Regular.woff) format('woff'),url(/katex-fonts/KaTeX_Size2-Regular.ttf) format('truetype');font-weight:normal;font-style:normal;}@font-face{font-family:'KaTeX_Size3';src:url(/katex-fonts/KaTeX_Size3-Regular.woff2) format('woff2'),url(/katex-fonts/KaTeX_Size3-Regular.woff) format('woff'),url(/katex-fonts/KaTeX_Size3-Regular.ttf) format('truetype');font-weight:normal;font-style:normal;}@font-face{font-family:'KaTeX_Size4';src:url(/katex-fonts/KaTeX_Size4-Regular.woff2) format('woff2'),url(/katex-fonts/KaTeX_Size4-Regular.woff) format('woff'),url(/katex-fonts/KaTeX_Size4-Regular.ttf) format('truetype');font-weight:normal;font-style:normal;}@font-face{font-family:'KaTeX_Typewriter';src:url(/katex-fonts/KaTeX_Typewriter-Regular.woff2) format('woff2'),url(/katex-fonts/KaTeX_Typewriter-Regular.woff) format('woff'),url(/katex-fonts/KaTeX_Typewriter-Regular.ttf) format('truetype');font-weight:normal;font-style:normal;}.css-4okk7a .katex{font:normal 1.21em KaTeX_Main,Times New Roman,serif;line-height:1.2;text-indent:0;text-rendering:auto;}.css-4okk7a .katex *{-ms-high-contrast-adjust:none!important;border-color:currentColor;}.css-4okk7a .katex .katex-version::after{content:'0.13.13';}.css-4okk7a .katex .katex-mathml{position:absolute;clip:rect(1px, 1px, 1px, 1px);padding:0;border:0;height:1px;width:1px;overflow:hidden;}.css-4okk7a .katex .katex-html>.newline{display:block;}.css-4okk7a .katex .base{position:relative;display:inline-block;white-space:nowrap;width:-webkit-min-content;width:-moz-min-content;width:-webkit-min-content;width:-moz-min-content;width:min-content;}.css-4okk7a .katex .strut{display:inline-block;}.css-4okk7a .katex .textbf{font-weight:bold;}.css-4okk7a .katex .textit{font-style:italic;}.css-4okk7a .katex .textrm{font-family:KaTeX_Main;}.css-4okk7a .katex .textsf{font-family:KaTeX_SansSerif;}.css-4okk7a .katex .texttt{font-family:KaTeX_Typewriter;}.css-4okk7a .katex .mathnormal{font-family:KaTeX_Math;font-style:italic;}.css-4okk7a .katex .mathit{font-family:KaTeX_Main;font-style:italic;}.css-4okk7a .katex .mathrm{font-style:normal;}.css-4okk7a .katex .mathbf{font-family:KaTeX_Main;font-weight:bold;}.css-4okk7a .katex .boldsymbol{font-family:KaTeX_Math;font-weight:bold;font-style:italic;}.css-4okk7a .katex .amsrm{font-family:KaTeX_AMS;}.css-4okk7a .katex .mathbb,.css-4okk7a .katex .textbb{font-family:KaTeX_AMS;}.css-4okk7a .katex .mathcal{font-family:KaTeX_Caligraphic;}.css-4okk7a .katex .mathfrak,.css-4okk7a .katex .textfrak{font-family:KaTeX_Fraktur;}.css-4okk7a .katex .mathtt{font-family:KaTeX_Typewriter;}.css-4okk7a .katex .mathscr,.css-4okk7a .katex .textscr{font-family:KaTeX_Script;}.css-4okk7a .katex .mathsf,.css-4okk7a .katex .textsf{font-family:KaTeX_SansSerif;}.css-4okk7a .katex .mathboldsf,.css-4okk7a .katex .textboldsf{font-family:KaTeX_SansSerif;font-weight:bold;}.css-4okk7a .katex .mathitsf,.css-4okk7a .katex .textitsf{font-family:KaTeX_SansSerif;font-style:italic;}.css-4okk7a .katex .mainrm{font-family:KaTeX_Main;font-style:normal;}.css-4okk7a .katex .vlist-t{display:inline-table;table-layout:fixed;border-collapse:collapse;}.css-4okk7a .katex .vlist-r{display:table-row;}.css-4okk7a .katex .vlist{display:table-cell;vertical-align:bottom;position:relative;}.css-4okk7a .katex .vlist>span{display:block;height:0;position:relative;}.css-4okk7a .katex .vlist>span>span{display:inline-block;}.css-4okk7a .katex .vlist>span>.pstrut{overflow:hidden;width:0;}.css-4okk7a .katex .vlist-t2{margin-right:-2px;}.css-4okk7a .katex .vlist-s{display:table-cell;vertical-align:bottom;font-size:1px;width:2px;min-width:2px;}.css-4okk7a .katex .vbox{display:-webkit-inline-box;display:-webkit-inline-flex;display:-ms-inline-flexbox;display:inline-flex;-webkit-flex-direction:column;-ms-flex-direction:column;flex-direction:column;-webkit-align-items:baseline;-webkit-box-align:baseline;-ms-flex-align:baseline;align-items:baseline;}.css-4okk7a .katex .hbox{display:-webkit-inline-box;display:-webkit-inline-flex;display:-ms-inline-flexbox;display:inline-flex;-webkit-flex-direction:row;-ms-flex-direction:row;flex-direction:row;width:100%;}.css-4okk7a .katex .thinbox{display:-webkit-inline-box;display:-webkit-inline-flex;display:-ms-inline-flexbox;display:inline-flex;-webkit-flex-direction:row;-ms-flex-direction:row;flex-direction:row;width:0;max-width:0;}.css-4okk7a .katex .msupsub{text-align:left;}.css-4okk7a .katex .mfrac>span>span{text-align:center;}.css-4okk7a .katex .mfrac .frac-line{display:inline-block;width:100%;border-bottom-style:solid;}.css-4okk7a .katex .mfrac .frac-line,.css-4okk7a .katex .overline .overline-line,.css-4okk7a .katex .underline .underline-line,.css-4okk7a .katex .hline,.css-4okk7a .katex .hdashline,.css-4okk7a .katex .rule{min-height:1px;}.css-4okk7a .katex .mspace{display:inline-block;}.css-4okk7a .katex .llap,.css-4okk7a .katex .rlap,.css-4okk7a .katex .clap{width:0;position:relative;}.css-4okk7a .katex .llap>.inner,.css-4okk7a .katex .rlap>.inner,.css-4okk7a .katex .clap>.inner{position:absolute;}.css-4okk7a .katex .llap>.fix,.css-4okk7a .katex .rlap>.fix,.css-4okk7a .katex .clap>.fix{display:inline-block;}.css-4okk7a .katex .llap>.inner{right:0;}.css-4okk7a .katex .rlap>.inner,.css-4okk7a .katex .clap>.inner{left:0;}.css-4okk7a .katex .clap>.inner>span{margin-left:-50%;margin-right:50%;}.css-4okk7a .katex .rule{display:inline-block;border:solid 0;position:relative;}.css-4okk7a .katex .overline .overline-line,.css-4okk7a .katex .underline .underline-line,.css-4okk7a .katex .hline{display:inline-block;width:100%;border-bottom-style:solid;}.css-4okk7a .katex .hdashline{display:inline-block;width:100%;border-bottom-style:dashed;}.css-4okk7a .katex .sqrt>.root{margin-left:0.27777778em;margin-right:-0.55555556em;}.css-4okk7a .katex .sizing.reset-size1.size1,.css-4okk7a .katex .fontsize-ensurer.reset-size1.size1{font-size:1em;}.css-4okk7a .katex .sizing.reset-size1.size2,.css-4okk7a .katex .fontsize-ensurer.reset-size1.size2{font-size:1.2em;}.css-4okk7a .katex .sizing.reset-size1.size3,.css-4okk7a .katex .fontsize-ensurer.reset-size1.size3{font-size:1.4em;}.css-4okk7a .katex .sizing.reset-size1.size4,.css-4okk7a .katex .fontsize-ensurer.reset-size1.size4{font-size:1.6em;}.css-4okk7a .katex .sizing.reset-size1.size5,.css-4okk7a .katex .fontsize-ensurer.reset-size1.size5{font-size:1.8em;}.css-4okk7a .katex .sizing.reset-size1.size6,.css-4okk7a .katex .fontsize-ensurer.reset-size1.size6{font-size:2em;}.css-4okk7a .katex .sizing.reset-size1.size7,.css-4okk7a .katex .fontsize-ensurer.reset-size1.size7{font-size:2.4em;}.css-4okk7a .katex .sizing.reset-size1.size8,.css-4okk7a .katex .fontsize-ensurer.reset-size1.size8{font-size:2.88em;}.css-4okk7a .katex .sizing.reset-size1.size9,.css-4okk7a .katex .fontsize-ensurer.reset-size1.size9{font-size:3.456em;}.css-4okk7a .katex .sizing.reset-size1.size10,.css-4okk7a .katex .fontsize-ensurer.reset-size1.size10{font-size:4.148em;}.css-4okk7a .katex .sizing.reset-size1.size11,.css-4okk7a .katex .fontsize-ensurer.reset-size1.size11{font-size:4.976em;}.css-4okk7a .katex .sizing.reset-size2.size1,.css-4okk7a .katex .fontsize-ensurer.reset-size2.size1{font-size:0.83333333em;}.css-4okk7a .katex .sizing.reset-size2.size2,.css-4okk7a .katex .fontsize-ensurer.reset-size2.size2{font-size:1em;}.css-4okk7a .katex .sizing.reset-size2.size3,.css-4okk7a .katex .fontsize-ensurer.reset-size2.size3{font-size:1.16666667em;}.css-4okk7a .katex .sizing.reset-size2.size4,.css-4okk7a .katex .fontsize-ensurer.reset-size2.size4{font-size:1.33333333em;}.css-4okk7a .katex .sizing.reset-size2.size5,.css-4okk7a .katex .fontsize-ensurer.reset-size2.size5{font-size:1.5em;}.css-4okk7a .katex .sizing.reset-size2.size6,.css-4okk7a .katex .fontsize-ensurer.reset-size2.size6{font-size:1.66666667em;}.css-4okk7a .katex .sizing.reset-size2.size7,.css-4okk7a .katex .fontsize-ensurer.reset-size2.size7{font-size:2em;}.css-4okk7a .katex .sizing.reset-size2.size8,.css-4okk7a .katex .fontsize-ensurer.reset-size2.size8{font-size:2.4em;}.css-4okk7a .katex .sizing.reset-size2.size9,.css-4okk7a .katex .fontsize-ensurer.reset-size2.size9{font-size:2.88em;}.css-4okk7a .katex .sizing.reset-size2.size10,.css-4okk7a .katex .fontsize-ensurer.reset-size2.size10{font-size:3.45666667em;}.css-4okk7a .katex .sizing.reset-size2.size11,.css-4okk7a .katex .fontsize-ensurer.reset-size2.size11{font-size:4.14666667em;}.css-4okk7a .katex .sizing.reset-size3.size1,.css-4okk7a .katex .fontsize-ensurer.reset-size3.size1{font-size:0.71428571em;}.css-4okk7a .katex .sizing.reset-size3.size2,.css-4okk7a .katex .fontsize-ensurer.reset-size3.size2{font-size:0.85714286em;}.css-4okk7a .katex .sizing.reset-size3.size3,.css-4okk7a .katex .fontsize-ensurer.reset-size3.size3{font-size:1em;}.css-4okk7a .katex .sizing.reset-size3.size4,.css-4okk7a .katex .fontsize-ensurer.reset-size3.size4{font-size:1.14285714em;}.css-4okk7a .katex .sizing.reset-size3.size5,.css-4okk7a .katex .fontsize-ensurer.reset-size3.size5{font-size:1.28571429em;}.css-4okk7a .katex .sizing.reset-size3.size6,.css-4okk7a .katex .fontsize-ensurer.reset-size3.size6{font-size:1.42857143em;}.css-4okk7a .katex .sizing.reset-size3.size7,.css-4okk7a .katex .fontsize-ensurer.reset-size3.size7{font-size:1.71428571em;}.css-4okk7a .katex .sizing.reset-size3.size8,.css-4okk7a .katex .fontsize-ensurer.reset-size3.size8{font-size:2.05714286em;}.css-4okk7a .katex .sizing.reset-size3.size9,.css-4okk7a .katex .fontsize-ensurer.reset-size3.size9{font-size:2.46857143em;}.css-4okk7a .katex .sizing.reset-size3.size10,.css-4okk7a .katex .fontsize-ensurer.reset-size3.size10{font-size:2.96285714em;}.css-4okk7a .katex .sizing.reset-size3.size11,.css-4okk7a .katex .fontsize-ensurer.reset-size3.size11{font-size:3.55428571em;}.css-4okk7a .katex .sizing.reset-size4.size1,.css-4okk7a .katex .fontsize-ensurer.reset-size4.size1{font-size:0.625em;}.css-4okk7a .katex .sizing.reset-size4.size2,.css-4okk7a .katex .fontsize-ensurer.reset-size4.size2{font-size:0.75em;}.css-4okk7a .katex .sizing.reset-size4.size3,.css-4okk7a .katex .fontsize-ensurer.reset-size4.size3{font-size:0.875em;}.css-4okk7a .katex .sizing.reset-size4.size4,.css-4okk7a .katex .fontsize-ensurer.reset-size4.size4{font-size:1em;}.css-4okk7a .katex .sizing.reset-size4.size5,.css-4okk7a .katex .fontsize-ensurer.reset-size4.size5{font-size:1.125em;}.css-4okk7a .katex .sizing.reset-size4.size6,.css-4okk7a .katex .fontsize-ensurer.reset-size4.size6{font-size:1.25em;}.css-4okk7a .katex .sizing.reset-size4.size7,.css-4okk7a .katex .fontsize-ensurer.reset-size4.size7{font-size:1.5em;}.css-4okk7a .katex .sizing.reset-size4.size8,.css-4okk7a .katex .fontsize-ensurer.reset-size4.size8{font-size:1.8em;}.css-4okk7a .katex .sizing.reset-size4.size9,.css-4okk7a .katex .fontsize-ensurer.reset-size4.size9{font-size:2.16em;}.css-4okk7a .katex .sizing.reset-size4.size10,.css-4okk7a .katex .fontsize-ensurer.reset-size4.size10{font-size:2.5925em;}.css-4okk7a .katex .sizing.reset-size4.size11,.css-4okk7a .katex .fontsize-ensurer.reset-size4.size11{font-size:3.11em;}.css-4okk7a .katex .sizing.reset-size5.size1,.css-4okk7a .katex .fontsize-ensurer.reset-size5.size1{font-size:0.55555556em;}.css-4okk7a .katex .sizing.reset-size5.size2,.css-4okk7a .katex .fontsize-ensurer.reset-size5.size2{font-size:0.66666667em;}.css-4okk7a .katex .sizing.reset-size5.size3,.css-4okk7a .katex .fontsize-ensurer.reset-size5.size3{font-size:0.77777778em;}.css-4okk7a .katex .sizing.reset-size5.size4,.css-4okk7a .katex .fontsize-ensurer.reset-size5.size4{font-size:0.88888889em;}.css-4okk7a .katex .sizing.reset-size5.size5,.css-4okk7a .katex .fontsize-ensurer.reset-size5.size5{font-size:1em;}.css-4okk7a .katex .sizing.reset-size5.size6,.css-4okk7a .katex .fontsize-ensurer.reset-size5.size6{font-size:1.11111111em;}.css-4okk7a .katex .sizing.reset-size5.size7,.css-4okk7a .katex .fontsize-ensurer.reset-size5.size7{font-size:1.33333333em;}.css-4okk7a .katex .sizing.reset-size5.size8,.css-4okk7a .katex .fontsize-ensurer.reset-size5.size8{font-size:1.6em;}.css-4okk7a .katex .sizing.reset-size5.size9,.css-4okk7a .katex .fontsize-ensurer.reset-size5.size9{font-size:1.92em;}.css-4okk7a .katex .sizing.reset-size5.size10,.css-4okk7a .katex .fontsize-ensurer.reset-size5.size10{font-size:2.30444444em;}.css-4okk7a .katex .sizing.reset-size5.size11,.css-4okk7a .katex .fontsize-ensurer.reset-size5.size11{font-size:2.76444444em;}.css-4okk7a .katex .sizing.reset-size6.size1,.css-4okk7a .katex .fontsize-ensurer.reset-size6.size1{font-size:0.5em;}.css-4okk7a .katex .sizing.reset-size6.size2,.css-4okk7a .katex .fontsize-ensurer.reset-size6.size2{font-size:0.6em;}.css-4okk7a .katex .sizing.reset-size6.size3,.css-4okk7a .katex .fontsize-ensurer.reset-size6.size3{font-size:0.7em;}.css-4okk7a .katex .sizing.reset-size6.size4,.css-4okk7a .katex .fontsize-ensurer.reset-size6.size4{font-size:0.8em;}.css-4okk7a .katex .sizing.reset-size6.size5,.css-4okk7a .katex .fontsize-ensurer.reset-size6.size5{font-size:0.9em;}.css-4okk7a .katex .sizing.reset-size6.size6,.css-4okk7a .katex .fontsize-ensurer.reset-size6.size6{font-size:1em;}.css-4okk7a .katex .sizing.reset-size6.size7,.css-4okk7a .katex .fontsize-ensurer.reset-size6.size7{font-size:1.2em;}.css-4okk7a .katex .sizing.reset-size6.size8,.css-4okk7a .katex .fontsize-ensurer.reset-size6.size8{font-size:1.44em;}.css-4okk7a .katex .sizing.reset-size6.size9,.css-4okk7a .katex .fontsize-ensurer.reset-size6.size9{font-size:1.728em;}.css-4okk7a .katex .sizing.reset-size6.size10,.css-4okk7a .katex .fontsize-ensurer.reset-size6.size10{font-size:2.074em;}.css-4okk7a .katex .sizing.reset-size6.size11,.css-4okk7a .katex .fontsize-ensurer.reset-size6.size11{font-size:2.488em;}.css-4okk7a .katex .sizing.reset-size7.size1,.css-4okk7a .katex .fontsize-ensurer.reset-size7.size1{font-size:0.41666667em;}.css-4okk7a .katex .sizing.reset-size7.size2,.css-4okk7a .katex .fontsize-ensurer.reset-size7.size2{font-size:0.5em;}.css-4okk7a .katex .sizing.reset-size7.size3,.css-4okk7a .katex .fontsize-ensurer.reset-size7.size3{font-size:0.58333333em;}.css-4okk7a .katex .sizing.reset-size7.size4,.css-4okk7a .katex .fontsize-ensurer.reset-size7.size4{font-size:0.66666667em;}.css-4okk7a .katex .sizing.reset-size7.size5,.css-4okk7a .katex .fontsize-ensurer.reset-size7.size5{font-size:0.75em;}.css-4okk7a .katex .sizing.reset-size7.size6,.css-4okk7a .katex .fontsize-ensurer.reset-size7.size6{font-size:0.83333333em;}.css-4okk7a .katex .sizing.reset-size7.size7,.css-4okk7a .katex .fontsize-ensurer.reset-size7.size7{font-size:1em;}.css-4okk7a .katex .sizing.reset-size7.size8,.css-4okk7a .katex .fontsize-ensurer.reset-size7.size8{font-size:1.2em;}.css-4okk7a .katex .sizing.reset-size7.size9,.css-4okk7a .katex .fontsize-ensurer.reset-size7.size9{font-size:1.44em;}.css-4okk7a .katex .sizing.reset-size7.size10,.css-4okk7a .katex .fontsize-ensurer.reset-size7.size10{font-size:1.72833333em;}.css-4okk7a .katex .sizing.reset-size7.size11,.css-4okk7a .katex .fontsize-ensurer.reset-size7.size11{font-size:2.07333333em;}.css-4okk7a .katex .sizing.reset-size8.size1,.css-4okk7a .katex .fontsize-ensurer.reset-size8.size1{font-size:0.34722222em;}.css-4okk7a .katex .sizing.reset-size8.size2,.css-4okk7a .katex .fontsize-ensurer.reset-size8.size2{font-size:0.41666667em;}.css-4okk7a .katex .sizing.reset-size8.size3,.css-4okk7a .katex .fontsize-ensurer.reset-size8.size3{font-size:0.48611111em;}.css-4okk7a .katex .sizing.reset-size8.size4,.css-4okk7a .katex .fontsize-ensurer.reset-size8.size4{font-size:0.55555556em;}.css-4okk7a .katex .sizing.reset-size8.size5,.css-4okk7a .katex .fontsize-ensurer.reset-size8.size5{font-size:0.625em;}.css-4okk7a .katex .sizing.reset-size8.size6,.css-4okk7a .katex .fontsize-ensurer.reset-size8.size6{font-size:0.69444444em;}.css-4okk7a .katex .sizing.reset-size8.size7,.css-4okk7a .katex .fontsize-ensurer.reset-size8.size7{font-size:0.83333333em;}.css-4okk7a .katex .sizing.reset-size8.size8,.css-4okk7a .katex .fontsize-ensurer.reset-size8.size8{font-size:1em;}.css-4okk7a .katex .sizing.reset-size8.size9,.css-4okk7a .katex .fontsize-ensurer.reset-size8.size9{font-size:1.2em;}.css-4okk7a .katex .sizing.reset-size8.size10,.css-4okk7a .katex .fontsize-ensurer.reset-size8.size10{font-size:1.44027778em;}.css-4okk7a .katex .sizing.reset-size8.size11,.css-4okk7a .katex .fontsize-ensurer.reset-size8.size11{font-size:1.72777778em;}.css-4okk7a .katex .sizing.reset-size9.size1,.css-4okk7a .katex .fontsize-ensurer.reset-size9.size1{font-size:0.28935185em;}.css-4okk7a .katex .sizing.reset-size9.size2,.css-4okk7a .katex .fontsize-ensurer.reset-size9.size2{font-size:0.34722222em;}.css-4okk7a .katex .sizing.reset-size9.size3,.css-4okk7a .katex .fontsize-ensurer.reset-size9.size3{font-size:0.40509259em;}.css-4okk7a .katex .sizing.reset-size9.size4,.css-4okk7a .katex .fontsize-ensurer.reset-size9.size4{font-size:0.46296296em;}.css-4okk7a .katex .sizing.reset-size9.size5,.css-4okk7a .katex .fontsize-ensurer.reset-size9.size5{font-size:0.52083333em;}.css-4okk7a .katex .sizing.reset-size9.size6,.css-4okk7a .katex .fontsize-ensurer.reset-size9.size6{font-size:0.5787037em;}.css-4okk7a .katex .sizing.reset-size9.size7,.css-4okk7a .katex .fontsize-ensurer.reset-size9.size7{font-size:0.69444444em;}.css-4okk7a .katex .sizing.reset-size9.size8,.css-4okk7a .katex .fontsize-ensurer.reset-size9.size8{font-size:0.83333333em;}.css-4okk7a .katex .sizing.reset-size9.size9,.css-4okk7a .katex .fontsize-ensurer.reset-size9.size9{font-size:1em;}.css-4okk7a .katex .sizing.reset-size9.size10,.css-4okk7a .katex .fontsize-ensurer.reset-size9.size10{font-size:1.20023148em;}.css-4okk7a .katex .sizing.reset-size9.size11,.css-4okk7a .katex .fontsize-ensurer.reset-size9.size11{font-size:1.43981481em;}.css-4okk7a .katex .sizing.reset-size10.size1,.css-4okk7a .katex .fontsize-ensurer.reset-size10.size1{font-size:0.24108004em;}.css-4okk7a .katex .sizing.reset-size10.size2,.css-4okk7a .katex .fontsize-ensurer.reset-size10.size2{font-size:0.28929605em;}.css-4okk7a .katex .sizing.reset-size10.size3,.css-4okk7a .katex .fontsize-ensurer.reset-size10.size3{font-size:0.33751205em;}.css-4okk7a .katex .sizing.reset-size10.size4,.css-4okk7a .katex .fontsize-ensurer.reset-size10.size4{font-size:0.38572806em;}.css-4okk7a .katex .sizing.reset-size10.size5,.css-4okk7a .katex .fontsize-ensurer.reset-size10.size5{font-size:0.43394407em;}.css-4okk7a .katex .sizing.reset-size10.size6,.css-4okk7a .katex .fontsize-ensurer.reset-size10.size6{font-size:0.48216008em;}.css-4okk7a .katex .sizing.reset-size10.size7,.css-4okk7a .katex .fontsize-ensurer.reset-size10.size7{font-size:0.57859209em;}.css-4okk7a .katex .sizing.reset-size10.size8,.css-4okk7a .katex .fontsize-ensurer.reset-size10.size8{font-size:0.69431051em;}.css-4okk7a .katex .sizing.reset-size10.size9,.css-4okk7a .katex .fontsize-ensurer.reset-size10.size9{font-size:0.83317261em;}.css-4okk7a .katex .sizing.reset-size10.size10,.css-4okk7a .katex .fontsize-ensurer.reset-size10.size10{font-size:1em;}.css-4okk7a .katex .sizing.reset-size10.size11,.css-4okk7a .katex .fontsize-ensurer.reset-size10.size11{font-size:1.19961427em;}.css-4okk7a .katex .sizing.reset-size11.size1,.css-4okk7a .katex .fontsize-ensurer.reset-size11.size1{font-size:0.20096463em;}.css-4okk7a .katex .sizing.reset-size11.size2,.css-4okk7a .katex .fontsize-ensurer.reset-size11.size2{font-size:0.24115756em;}.css-4okk7a .katex .sizing.reset-size11.size3,.css-4okk7a .katex .fontsize-ensurer.reset-size11.size3{font-size:0.28135048em;}.css-4okk7a .katex .sizing.reset-size11.size4,.css-4okk7a .katex .fontsize-ensurer.reset-size11.size4{font-size:0.32154341em;}.css-4okk7a .katex .sizing.reset-size11.size5,.css-4okk7a .katex .fontsize-ensurer.reset-size11.size5{font-size:0.36173633em;}.css-4okk7a .katex .sizing.reset-size11.size6,.css-4okk7a .katex .fontsize-ensurer.reset-size11.size6{font-size:0.40192926em;}.css-4okk7a .katex .sizing.reset-size11.size7,.css-4okk7a .katex .fontsize-ensurer.reset-size11.size7{font-size:0.48231511em;}.css-4okk7a .katex .sizing.reset-size11.size8,.css-4okk7a .katex .fontsize-ensurer.reset-size11.size8{font-size:0.57877814em;}.css-4okk7a .katex .sizing.reset-size11.size9,.css-4okk7a .katex .fontsize-ensurer.reset-size11.size9{font-size:0.69453376em;}.css-4okk7a .katex .sizing.reset-size11.size10,.css-4okk7a .katex .fontsize-ensurer.reset-size11.size10{font-size:0.83360129em;}.css-4okk7a .katex .sizing.reset-size11.size11,.css-4okk7a .katex .fontsize-ensurer.reset-size11.size11{font-size:1em;}.css-4okk7a .katex .delimsizing.size1{font-family:KaTeX_Size1;}.css-4okk7a .katex .delimsizing.size2{font-family:KaTeX_Size2;}.css-4okk7a .katex .delimsizing.size3{font-family:KaTeX_Size3;}.css-4okk7a .katex .delimsizing.size4{font-family:KaTeX_Size4;}.css-4okk7a .katex .delimsizing.mult .delim-size1>span{font-family:KaTeX_Size1;}.css-4okk7a .katex .delimsizing.mult .delim-size4>span{font-family:KaTeX_Size4;}.css-4okk7a .katex .nulldelimiter{display:inline-block;width:0.12em;}.css-4okk7a .katex .delimcenter{position:relative;}.css-4okk7a .katex .op-symbol{position:relative;}.css-4okk7a .katex .op-symbol.small-op{font-family:KaTeX_Size1;}.css-4okk7a .katex .op-symbol.large-op{font-family:KaTeX_Size2;}.css-4okk7a .katex .op-limits>.vlist-t{text-align:center;}.css-4okk7a .katex .accent>.vlist-t{text-align:center;}.css-4okk7a .katex .accent .accent-body{position:relative;}.css-4okk7a .katex .accent .accent-body:not(.accent-full){width:0;}.css-4okk7a .katex .overlay{display:block;}.css-4okk7a .katex .mtable .vertical-separator{display:inline-block;min-width:1px;}.css-4okk7a .katex .mtable .arraycolsep{display:inline-block;}.css-4okk7a .katex .mtable .col-align-c>.vlist-t{text-align:center;}.css-4okk7a .katex .mtable .col-align-l>.vlist-t{text-align:left;}.css-4okk7a .katex .mtable .col-align-r>.vlist-t{text-align:right;}.css-4okk7a .katex .svg-align{text-align:left;}.css-4okk7a .katex svg{display:block;position:absolute;width:100%;height:inherit;fill:currentColor;stroke:currentColor;fill-rule:nonzero;fill-opacity:1;stroke-width:1;stroke-linecap:butt;stroke-linejoin:miter;stroke-miterlimit:4;stroke-dasharray:none;stroke-dashoffset:0;stroke-opacity:1;}.css-4okk7a .katex svg path{stroke:none;}.css-4okk7a .katex img{border-style:none;min-width:0;min-height:0;max-width:none;max-height:none;}.css-4okk7a .katex .stretchy{width:100%;display:block;position:relative;overflow:hidden;}.css-4okk7a .katex .stretchy::before,.css-4okk7a .katex .stretchy::after{content:'';}.css-4okk7a .katex .hide-tail{width:100%;position:relative;overflow:hidden;}.css-4okk7a .katex .halfarrow-left{position:absolute;left:0;width:50.2%;overflow:hidden;}.css-4okk7a .katex .halfarrow-right{position:absolute;right:0;width:50.2%;overflow:hidden;}.css-4okk7a .katex .brace-left{position:absolute;left:0;width:25.1%;overflow:hidden;}.css-4okk7a .katex .brace-center{position:absolute;left:25%;width:50%;overflow:hidden;}.css-4okk7a .katex .brace-right{position:absolute;right:0;width:25.1%;overflow:hidden;}.css-4okk7a .katex .x-arrow-pad{padding:0 0.5em;}.css-4okk7a .katex .cd-arrow-pad{padding:0 0.55556em 0 0.27778em;}.css-4okk7a .katex .x-arrow,.css-4okk7a .katex .mover,.css-4okk7a .katex .munder{text-align:center;}.css-4okk7a .katex .boxpad{padding:0 0.3em 0 0.3em;}.css-4okk7a .katex .fbox,.css-4okk7a .katex .fcolorbox{box-sizing:border-box;border:0.04em solid;}.css-4okk7a .katex .cancel-pad{padding:0 0.2em 0 0.2em;}.css-4okk7a .katex .cancel-lap{margin-left:-0.2em;margin-right:-0.2em;}.css-4okk7a .katex .sout{border-bottom-style:solid;border-bottom-width:0.08em;}.css-4okk7a .katex .angl{box-sizing:border-box;border-top:0.049em solid;border-right:0.049em solid;margin-right:0.03889em;}.css-4okk7a .katex .anglpad{padding:0 0.03889em 0 0.03889em;}.css-4okk7a .katex .eqn-num::before{counter-increment:katexEqnNo;content:'(' counter(katexEqnNo) ')';}.css-4okk7a .katex .mml-eqn-num::before{counter-increment:mmlEqnNo;content:'(' counter(mmlEqnNo) ')';}.css-4okk7a .katex .mtr-glue{width:50%;}.css-4okk7a .katex .cd-vert-arrow{display:inline-block;position:relative;}.css-4okk7a .katex .cd-label-left{display:inline-block;position:absolute;right:calc(50% + 0.3em);text-align:left;}.css-4okk7a .katex .cd-label-right{display:inline-block;position:absolute;left:calc(50% + 0.3em);text-align:right;}.css-4okk7a .katex-display{display:block;margin:1em 0;text-align:center;}.css-4okk7a .katex-display>.katex{display:block;white-space:nowrap;}.css-4okk7a .katex-display>.katex>.katex-html{display:block;position:relative;}.css-4okk7a .katex-display>.katex>.katex-html>.tag{position:absolute;right:0;}.css-4okk7a .katex-display.leqno>.katex>.katex-html>.tag{left:0;right:auto;}.css-4okk7a .katex-display.fleqn>.katex{text-align:left;padding-left:2em;}.css-4okk7a body{counter-reset:katexEqnNo mmlEqnNo;}.css-4okk7a table{width:-webkit-max-content;width:-moz-max-content;width:max-content;}.css-4okk7a .tableBlock{max-width:100%;margin-bottom:1rem;overflow-y:scroll;}.css-4okk7a .tableBlock thead,.css-4okk7a .tableBlock thead th{border-bottom:1px solid #333!important;}.css-4okk7a .tableBlock th,.css-4okk7a .tableBlock td{padding:10px;text-align:left;}.css-4okk7a .tableBlock th{font-weight:bold!important;}.css-4okk7a .tableBlock caption{caption-side:bottom;color:#555;font-size:12px;font-style:italic;text-align:center;}.css-4okk7a .tableBlock caption>p{margin:0;}.css-4okk7a .tableBlock th>p,.css-4okk7a .tableBlock td>p{margin:0;}.css-4okk7a .tableBlock [data-background-color='aliceblue']{background-color:#f0f8ff;color:#000;}.css-4okk7a .tableBlock [data-background-color='black']{background-color:#000;color:#fff;}.css-4okk7a .tableBlock [data-background-color='chocolate']{background-color:#d2691e;color:#fff;}.css-4okk7a .tableBlock [data-background-color='cornflowerblue']{background-color:#6495ed;color:#fff;}.css-4okk7a .tableBlock [data-background-color='crimson']{background-color:#dc143c;color:#fff;}.css-4okk7a .tableBlock [data-background-color='darkblue']{background-color:#00008b;color:#fff;}.css-4okk7a .tableBlock [data-background-color='darkseagreen']{background-color:#8fbc8f;color:#000;}.css-4okk7a .tableBlock [data-background-color='deepskyblue']{background-color:#00bfff;color:#000;}.css-4okk7a .tableBlock [data-background-color='gainsboro']{background-color:#dcdcdc;color:#000;}.css-4okk7a .tableBlock [data-background-color='grey']{background-color:#808080;color:#fff;}.css-4okk7a .tableBlock [data-background-color='lemonchiffon']{background-color:#fffacd;color:#000;}.css-4okk7a .tableBlock [data-background-color='lightpink']{background-color:#ffb6c1;color:#000;}.css-4okk7a .tableBlock [data-background-color='lightsalmon']{background-color:#ffa07a;color:#000;}.css-4okk7a .tableBlock [data-background-color='lightskyblue']{background-color:#87cefa;color:#000;}.css-4okk7a .tableBlock [data-background-color='mediumblue']{background-color:#0000cd;color:#fff;}.css-4okk7a .tableBlock [data-background-color='omnigrey']{background-color:#f0f0f0;color:#000;}.css-4okk7a .tableBlock [data-background-color='white']{background-color:#fff;color:#000;}.css-4okk7a .tableBlock [data-text-align='center']{text-align:center;}.css-4okk7a .tableBlock [data-text-align='left']{text-align:left;}.css-4okk7a .tableBlock [data-text-align='right']{text-align:right;}.css-4okk7a .tableBlock [data-vertical-align='bottom']{vertical-align:bottom;}.css-4okk7a .tableBlock [data-vertical-align='middle']{vertical-align:middle;}.css-4okk7a .tableBlock [data-vertical-align='top']{vertical-align:top;}.css-4okk7a .tableBlock__font-size--xxsmall{font-size:10px;}.css-4okk7a .tableBlock__font-size--xsmall{font-size:12px;}.css-4okk7a .tableBlock__font-size--small{font-size:14px;}.css-4okk7a .tableBlock__font-size--large{font-size:18px;}.css-4okk7a .tableBlock__border--some tbody tr:not(:last-child){border-bottom:1px solid #e2e5e7;}.css-4okk7a .tableBlock__border--bordered td,.css-4okk7a .tableBlock__border--bordered th{border:1px solid #e2e5e7;}.css-4okk7a .tableBlock__border--borderless tbody+tbody,.css-4okk7a .tableBlock__border--borderless td,.css-4okk7a .tableBlock__border--borderless th,.css-4okk7a .tableBlock__border--borderless tr,.css-4okk7a .tableBlock__border--borderless thead,.css-4okk7a .tableBlock__border--borderless thead th{border:0!important;}.css-4okk7a .tableBlock:not(.tableBlock__table-striped) tbody tr{background-color:unset!important;}.css-4okk7a .tableBlock__table-striped tbody tr:nth-of-type(odd){background-color:#f9fafc!important;}.css-4okk7a .tableBlock__table-compactl th,.css-4okk7a .tableBlock__table-compact td{padding:3px!important;}.css-4okk7a .tableBlock__full-size{width:100%;}.css-4okk7a .textBlock{margin-bottom:16px;}.css-4okk7a .textBlock__text-formatting--finePrint{font-size:12px;}.css-4okk7a .textBlock__text-infoBox{padding:0.75rem 1.25rem;margin-bottom:1rem;border:1px solid transparent;border-radius:0.25rem;}.css-4okk7a .textBlock__text-infoBox p{margin:0;}.css-4okk7a .textBlock__text-infoBox--primary{background-color:#cce5ff;border-color:#b8daff;color:#004085;}.css-4okk7a .textBlock__text-infoBox--secondary{background-color:#e2e3e5;border-color:#d6d8db;color:#383d41;}.css-4okk7a .textBlock__text-infoBox--success{background-color:#d4edda;border-color:#c3e6cb;color:#155724;}.css-4okk7a .textBlock__text-infoBox--danger{background-color:#f8d7da;border-color:#f5c6cb;color:#721c24;}.css-4okk7a .textBlock__text-infoBox--warning{background-color:#fff3cd;border-color:#ffeeba;color:#856404;}.css-4okk7a .textBlock__text-infoBox--info{background-color:#d1ecf1;border-color:#bee5eb;color:#0c5460;}.css-4okk7a .textBlock__text-infoBox--dark{background-color:#d6d8d9;border-color:#c6c8ca;color:#1b1e21;}.css-4okk7a .text-overline{-webkit-text-decoration:overline;text-decoration:overline;}.css-4okk7a.css-4okk7a{color:#2B3148;background-color:transparent;font-family:"Roboto","Helvetica","Arial",sans-serif;font-size:20px;line-height:24px;overflow:visible;padding-top:0px;position:relative;}.css-4okk7a.css-4okk7a:after{content:'';-webkit-transform:scale(0);-moz-transform:scale(0);-ms-transform:scale(0);transform:scale(0);position:absolute;border:2px solid #EA9430;border-radius:2px;inset:-8px;z-index:1;}.css-4okk7a .js-external-link-button.link-like,.css-4okk7a .js-external-link-anchor{color:inherit;border-radius:1px;-webkit-text-decoration:underline;text-decoration:underline;}.css-4okk7a .js-external-link-button.link-like:hover,.css-4okk7a .js-external-link-anchor:hover,.css-4okk7a .js-external-link-button.link-like:active,.css-4okk7a .js-external-link-anchor:active{text-decoration-thickness:2px;text-shadow:1px 0 0;}.css-4okk7a .js-external-link-button.link-like:focus-visible,.css-4okk7a .js-external-link-anchor:focus-visible{outline:transparent 2px dotted;box-shadow:0 0 0 2px #6314E6;}.css-4okk7a p,.css-4okk7a div{margin:0px;display:block;}.css-4okk7a pre{margin:0px;display:block;}.css-4okk7a pre code{display:block;width:-webkit-fit-content;width:-moz-fit-content;width:fit-content;}.css-4okk7a pre:not(:first-child){padding-top:8px;}.css-4okk7a ul,.css-4okk7a ol{display:block margin:0px;padding-left:20px;}.css-4okk7a ul li,.css-4okk7a ol li{padding-top:8px;}.css-4okk7a ul ul,.css-4okk7a ol ul,.css-4okk7a ul ol,.css-4okk7a ol ol{padding-top:0px;}.css-4okk7a ul:not(:first-child),.css-4okk7a ol:not(:first-child){padding-top:4px;} Speed of sound
Speed of Sound Calculator
Calculator for determining the time it takes for sound to travel a distance and for the distance traveled. Helpful, e.g. during a thunderstorm , where lightning can be seen first and then thunder can be heard a few seconds later. The speed of sound at 20°C and high humidity is approx. 343 m/s (= 1235 km/h), at cooler temperatures and low humidity it is a little bit lower. Enter the duration or the distance, the other value will be calculated. Or calculate the speed of sound by deleting the value there and entering duration and distance.
Physics commonly uses SI units. Here is a calculator to convert lengths , temperature , pressure , speed and other units.
Want to create or adapt books like this? Learn more about how Pressbooks supports open publishing practices.
Traveling waves
17 How sound moves
Speed of sound.
There’s a delay between when a sound is created and when it is heard. In everyday life, the delay is usually too short to notice. However, the delay can be noticeable if the distance between source and detector is large enough. You see lightning before you hear the thunder. If you’ve sat in the outfield seats in a baseball stadium, you’ve experienced the delay between seeing the player hit the ball and the sound of the “whack.” Life experiences tell us that sound travels fast, but not nearly as fast as light does. Careful experiments confirm this idea.
The speed of sound in air is roughly 340 m/s. The actual value depends somewhat on the temperature and humidity. In everyday terms, sound travels about the length of three and a half foot ball fields every second- about 50% faster than a Boeing 747 (roughly 250 m/s). This may seem fast, but it’s tiny compared to light, which travels roughly a million times faster than sound (roughly 300,000,000 m/s).
Sound requires some material in which to propagate (i.e. travel). This material sound travels through is called the medium . You can show that sound requires a medium by putting a cell phone inside a glass jar connected to a vacuum pump. As the air is removed from the jar, the cell phone’s ringer gets quieter and quieter. A youTube video (2:05 min) produced by the UNSW PhysClips project shows the demo with a drumming toy monkey [1] instead of a cell phone.
What affects the speed of sound?
Sound travels at different speeds though different materials. The physical properties of the medium are the only factors that affect the speed of sound- nothing else matters.
The speed of sound in a material is determined mainly by two properties- the stiffness of the material and the density of the material. Sound travels fastest through materials that are stiff and light. In general, sound travels fastest through solids, slower through liquids and slowest through gasses. (See the table on this page). This may seem backwards- after all, metals are quite dense. However, the high density of metals is more than offset by far greater stiffness (compared to liquids and solids).
The speed of sound in air depends mainly on temperature. The speed of sound is 331 m/s in dry air at 0 o Celsius and increases slightly with temperature- about 0.6 m/s for every 1 o Celsius for temperatures commonly found on Earth. Though speed of sound in air also depends on humidity, the effect is tiny- sound travels only about 1 m/s faster in air with 100% humidity air at 20 o C than it does in completely dry air at the same temperature.
Nothing else matters
The properties of the medium are the only factors that affect the speed of sound- nothing else matters.
Frequency of the sound does not matter- high frequency sounds travel at the same speed as low frequency sounds. If you’ve ever listened to music, you’ve witnessed this- the low notes and the high notes that were made simultaneously reach you simultaneously, even if you are far from the stage. If you’ve heard someone shout from across a field, you’ve noticed that the entire shout sound (which contains many different frequencies at once) reaches you at the same time. If different frequencies traveled at different rates, some frequencies would arrive before others.
The amplitude of the sound does not matter- loud sounds and quiet ones travel at the same speed. Whisper or yell- it doesn’t matter. The sound still takes the same amount of time to reach the listener. You’ve probably heard that you can figure out how far away the lightning by counting the seconds between when you see lightning and hear thunder. If the speed of sound depended on loudness, this rule of thumb would have to account for loudness- yet there is nothing in the rule about loud vs. quiet thunder. The rule of thumb works the same for all thunder- regardless of loudness . That’s because the speed of sound doesn’t depend on amplitude.
Stop to thinks
- Which takes longer to cross a football field: the sound of a high pitched chirp emitted by a fruit bat or the (relatively) low pitched sound emitted by a trumpet?
- Which sound takes longer to travel 100 meters: the sound of a snapping twig in the forest or the sound of a gunshot?
- Which takes longer to travel the distance of a football field: the low pitched sound of a whale or the somewhat higher pitched sound of a human being?
Constant speed
Sound travels at a constant speed. Sound does not speed up or slow down as it travels (unless the properties of the material the sound is going through changes). I know what you’re thinking- how is that possible? Sounds die out as they travel, right? True. That means sounds must slow down and come to a stop, right? Wrong. As sound travels, its amplitude decreases- but that’s not the same thing as slowing down. Sound (in air) covers roughly 340 meters each and every second, even as its amplitude shrinks. Eventually, the amplitude gets small enough that the sound is undetectable. A sound’s amplitude shrinks as it travels, but its speed remains constant.
The basic equation for constant speed motion (shown below) applies to sound.
[latex]d=vt[/latex]
In this equation, [latex]d[/latex] represents the distance traveled by the sound, [latex]t[/latex] represents the amount of time it took to go that distance and [latex]v[/latex] represents the speed.
Rule of thumb for lightning example
Example: thunder and lightning.
The rule of thumb for figuring out how far away a lightning strike is from you is this:
Count the number of seconds between when you see the lightning and hear the thunder. Divide the number of seconds by five to find out how many miles away the lightning hit.
According to this rule, what is the speed of sound in air? How does the speed of sound implied by this rule compare to 340 m/s?
Identify important physics concept : This question is about speed of sound.
List known and unknown quantities (with letter names and units):
At first glance, it doesn’t look like there’s enough information to solve the problem. We were asked to find speed, but not given either a time or a distance. However, the problem does allow us to figure out a distance if we know the time- “Divide the number of seconds by five to find out how many miles away the lightning hit.” So, let’s make up a time and see what happens; if the time is 10 seconds, the rule of thumb says that the distance should be 2 miles.
[latex]v= \: ?[/latex]
[latex]d=2 \: miles[/latex]
[latex]t=10 \: seconds[/latex]
You might ask “Is making stuff up OK here?” The answer is YES! If the rule of thumb is right, it should work no matter what time we choose. (To check if the rule is OK, we should probably test it with more than just one distance-time combination, but we’ll assume the rule is OK for now).
Do the algebra: The equation is already solved for speed. Move on to the next step.
Do unit conversions (if needed) then plug in numbers: If you just plug in the numbers, the speed comes out in miles per second:
[latex]v = \frac{2 \: miles} {10 \: seconds}=0.2 \: \frac{miles} {second}[/latex]
We are asked to compare this speed to 340 m/s, so a unit conversion is in order; since there are 1609 meters in a mile:
[latex]v =0.2 \: \frac{miles} {second}*\frac{1609 \: meters} {1 \:mile}=320 \frac{m}{s}[/latex]
Reflect on the answer:
- The answer for speed from the rule of thumb is less than 10% off the actual value of roughly 340 m/s- surprisingly close!
- At the beginning, we assumed a time of 10 seconds. Does the result hold up for other choices? A quick check shows that it does! For instance, if we use a time of 5 seconds, the rule of thumb gives a distance of 1 mile, and the math still gives a speed of 0.2 miles/second. The speed will be the same no matter what time we pick. The reason is this: The more time it takes the thunder to arrive, the farther away the lightning strike is, but the speed remains the same. In the equation for speed, both time and distance change by the same factor and the overall fraction remains unchanged.
Stop to think answers
- Both sounds take the same amount of time. (High and low pitched sounds travel at the same speed).
- Both sounds take the same amount of time. (Quiet sounds and loud sounds travel at the same speed).
- The sound of the whale travels the distance in less time- assuming sound from the whale travels in water and sound from the human travels in air. Sound travels faster in water than in air. (The info about frequency was given just to throw you off- frequency doesn’t matter).
- Wolfe, J. (2014, February 20). Properties of Sound. Retrieved from https://www.youtube.com/watch?v=P8-govgAffY ↵
Understanding Sound Copyright © by dsa2gamba and abbottds is licensed under a Creative Commons Attribution 4.0 International License , except where otherwise noted.
Share This Book

- Rivers and Lakes
- Severe Weather
- Fire Weather
- Long Range Forecasts
- Climate Prediction
- Space Weather
- Past Weather
- Heating/Cooling Days
- Monthly Temperatures
- Astronomical Data
- Beach Hazards
- Air Quality
- Safe Boating
- Rip Currents
- Thunderstorms
- Sun (Ultraviolet Radiation)
- Safety Campaigns
- Winter Weather
- Wireless Emergency Alerts
- Weather-Ready Nation
- Cooperative Observers
- Daily Briefing
- Damage/Fatality/Injury Statistics
- Forecast Models
- GIS Data Portal
- NOAA Weather Radio
- Publications
- SKYWARN Storm Spotters
- TsunamiReady
- Service Change Notices
- Be A Force of Nature
- NWS Education Home
- Pubs/Brochures/Booklets
- NWS Media Contacts
NWS All NOAA
- Organization
- Strategic Plan
- Commitment to Diversity
- For NWS Employees
- International
- National Centers
- Social Media
National Program
Understanding Lightning: Thunder
Thunder is the sound caused by a nearby flash of lightning and can be heard for a distance of only about 10 miles from the lightning strike. The sound of thunder should serve as a warning to anyone outside that they are within striking distance of the storm and need to get to a safe place immediately!
Thunder is created when lightning passes through the air. The lightning discharge heats the air rapidly and causes it to expand. The temperature of the air in the lightning channel may reach as high as 50,000 degrees Fahrenheit, 5 times hotter than the surface of the sun. Immediately after the flash, the air cools and contracts quickly. This rapid expansion and contraction creates the sound wave that we hear as thunder.
Although a lightning discharge usually strikes just one spot on the ground, it travels many miles through the air. When you listen to thunder, you'll first hear the thunder created by that portion of the lightning channel that is nearest you. As you continue to listen, you'll hear the sound created from the portions of the channel farther and farther away. Typically, a sharp crack or click will indicate that the lightning channel passed nearby. If the thunder sounds more like a rumble, the lightning was at least several miles away. The loud boom that you sometimes hear is created by the main lightning channel as it reaches the ground.
Since you see lightning immediately and it takes the sound of thunder about 5 seconds to travel a mile, you can calculate the distance between you and the lightning. If you count the number of seconds between the flash of lightning and the sound of thunder, and then divide by 5, you'll get the distance in miles to the lightning: 5 seconds = 1 mile, 15 seconds = 3 miles, 0 seconds = very close.
Keep in mind that you should be in a safe place while counting. Remember, if you can hear thunder, chances are that you're within striking distance of the storm. You don't want to get struck by the next flash of lightning.
To learn more, see Acknowledgements and References or return to Contents page

US Dept of Commerce National Oceanic and Atmospheric Administration National Weather Service Safety 1325 East West Highway Silver Spring, MD 20910 Comments? Questions? Please Contact Us.
Related Resources: calculators
Distance to Lightening Strike Formula and Calculator
Engineering Physics
When lightening strikes sound is created at that moment. Since light travels extremely fast and is undetectable (186,000 miles/sec. or 671,000,000 miles/hour) to human perception we can use the speed of sound to estimate the distance between the lightening strike and our location.
On a typical day at sea level sound travels at 761.1 mph. As our altitude increases there are changes in air density as well as temperature and the speed of sound also changes, see
Speed of Sound Table and Formula
Thus, the time required for sound to travel one mile is:
time (seconds) = ( 761.1 mile/hr) * (1 hr / 3,600 sec) time = 0.2114 seconds
thus the speed of sound can also be stated as 0.2114 miles/sec
Distance to lightening = time (sec.) * 0.2114 miles / sec.
For simplicity and estimating the distance from the lightening strike over your favorite brew we typically round up to 5 seconds per mile from the lightening flash.
Therefore if you estimate 5 seconds from the flash of lightening to hearing the thunder the lightening struck approximately one mile away.
National Weather Service Nist
Link to this Webpage :
Copy Text to clipboard
© Copyright 2000 - 2024, by Engineers Edge, LLC www.engineersedge.com All rights reserved Disclaimer | Feedback Advertising | Contact
- Random article
- Teaching guide
- Privacy & cookies
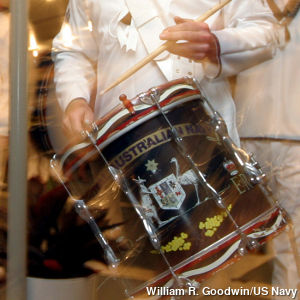
by Chris Woodford . Last updated: July 23, 2023.
Photo: Sound is energy we hear made by things that vibrate. Photo by William R. Goodwin courtesy of US Navy and Wikimedia Commons .
What is sound?
Photo: Sensing with sound: Light doesn't travel well through ocean water: over half the light falling on the sea surface is absorbed within the first meter of water; 100m down and only 1 percent of the surface light remains. That's largely why mighty creatures of the deep rely on sound for communication and navigation. Whales, famously, "talk" to one another across entire ocean basins, while dolphins use sound, like bats, for echolocation. Photo by Bill Thompson courtesy of US Fish and Wildlife Service .
Robert Boyle's classic experiment
Artwork: Robert Boyle's famous experiment with an alarm clock.
How sound travels
Artwork: Sound waves and ocean waves compared. Top: Sound waves are longitudinal waves: the air moves back and forth along the same line as the wave travels, making alternate patterns of compressions and rarefactions. Bottom: Ocean waves are transverse waves: the water moves back and forth at right angles to the line in which the wave travels.
The science of sound waves
Picture: Reflected sound is extremely useful for "seeing" underwater where light doesn't really travel—that's the basic idea behind sonar. Here's a side-scan sonar (reflected sound) image of a World War II boat wrecked on the seabed. Photo courtesy of U.S. National Oceanographic and Atmospheric Administration, US Navy, and Wikimedia Commons .
Whispering galleries and amphitheaters
Photos by Carol M. Highsmith: 1) The Capitol in Washington, DC has a whispering gallery inside its dome. Photo credit: The George F. Landegger Collection of District of Columbia Photographs in Carol M. Highsmith's America, Library of Congress , Prints and Photographs Division. 2) It's easy to hear people talking in the curved memorial amphitheater building at Arlington National Cemetery, Arlington, Virginia. Photo credit: Photographs in the Carol M. Highsmith Archive, Library of Congress , Prints and Photographs Division.
Measuring waves
Understanding amplitude and frequency, why instruments sound different, the speed of sound.
Photo: Breaking through the sound barrier creates a sonic boom. The mist you can see, which is called a condensation cloud, isn't necessarily caused by an aircraft flying supersonic: it can occur at lower speeds too. It happens because moist air condenses due to the shock waves created by the plane. You might expect the plane to compress the air as it slices through. But the shock waves it generates alternately expand and contract the air, producing both compressions and rarefactions. The rarefactions cause very low pressure and it's these that make moisture in the air condense, producing the cloud you see here. Photo by John Gay courtesy of US Navy and Wikimedia Commons .
Why does sound go faster in some things than in others?
Chart: Generally, sound travels faster in solids (right) than in liquids (middle) or gases (left)... but there are exceptions!
How to measure the speed of sound
Sound in practice, if you liked this article..., find out more, on this website.
- Electric guitars
- Speech synthesis
- Synthesizers
On other sites
- Explore Sound : A comprehensive educational site from the Acoustical Society of America, with activities for students of all ages.
- Sound Waves : A great collection of interactive science lessons from the University of Salford, which explains what sound waves are and the different ways in which they behave.
Educational books for younger readers
- Sound (Science in a Flash) by Georgia Amson-Bradshaw. Franklin Watts/Hachette, 2020. Simple facts, experiments, and quizzes fill this book; the visually exciting design will appeal to reluctant readers. Also for ages 7–9.
- Sound by Angela Royston. Raintree, 2017. A basic introduction to sound and musical sounds, including simple activities. Ages 7–9.
- Experimenting with Sound Science Projects by Robert Gardner. Enslow Publishers, 2013. A comprehensive 120-page introduction, running through the science of sound in some detail, with plenty of hands-on projects and activities (including welcome coverage of how to run controlled experiments using the scientific method). Ages 9–12.
- Cool Science: Experiments with Sound and Hearing by Chris Woodford. Gareth Stevens Inc, 2010. One of my own books, this is a short introduction to sound through practical activities, for ages 9–12.
- Adventures in Sound with Max Axiom, Super Scientist by Emily Sohn. Capstone, 2007. The original, graphic novel (comic book) format should appeal to reluctant readers. Ages 8–10.
Popular science
- The Sound Book: The Science of the Sonic Wonders of the World by Trevor Cox. W. W. Norton, 2014. An entertaining tour through everyday sound science.
Academic books
- Master Handbook of Acoustics by F. Alton Everest and Ken Pohlmann. McGraw-Hill Education, 2015. A comprehensive reference for undergraduates and sound-design professionals.
- The Science of Sound by Thomas D. Rossing, Paul A. Wheeler, and F. Richard Moore. Pearson, 2013. One of the most popular general undergraduate texts.
Text copyright © Chris Woodford 2009, 2021. All rights reserved. Full copyright notice and terms of use .
Rate this page
Tell your friends, cite this page, more to explore on our website....
- Get the book
- Send feedback
The Enlightened Mindset
Exploring the World of Knowledge and Understanding
Welcome to the world's first fully AI generated website!
How Long Does it Take Sound to Travel 1 Mile? An Exploration of the Speed of Sound
By Happy Sharer
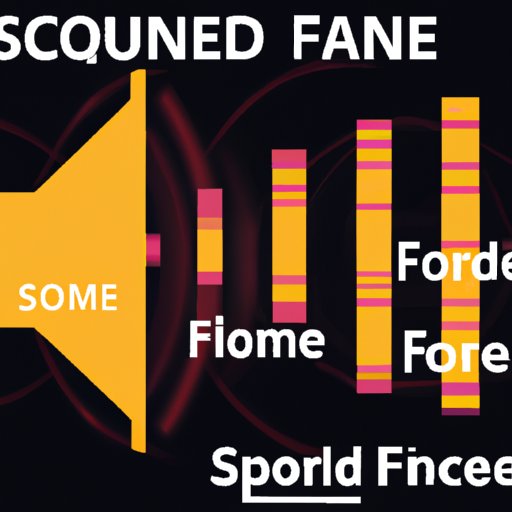
Introduction
Sound is a wave of energy created by vibrating objects and transmitted through a medium such as air or water. The speed of sound is determined by the type of medium it travels through and is measured in meters per second (m/s). Depending on the environment, sound can travel at different speeds, making it difficult to determine exactly how long it takes for sound to cover a certain distance.
In this article, we will explore how long it takes for sound to travel one mile and what factors influence the speed of sound. We will look at the different ways to measure sound over a mile, as well as calculations that can help us determine the exact time it takes for sound to reach one mile.
Exploring the Speed of Sound: How Long Does it Take for Sound to Travel 1 Mile?
The speed of sound is affected by various factors, including the type of medium it is travelling through and the temperature of the environment. In general, sound travels faster in warmer environments than in colder ones. For example, sound travels at 343 m/s in air at 0°C, but can reach up to 369 m/s at 30°C.
In order to determine how long it takes for sound to travel one mile, we need to calculate the time it takes for sound to cover a certain distance. To do this, we divide the distance (in meters) by the speed of sound (in m/s). For example, if the distance is 1609.344 meters (1 mile) and the speed of sound is 343 m/s, it would take 4.7 seconds for sound to cover one mile.
What’s the Rush? Measuring the Time it Takes for Sound to Travel One Mile
The speed of sound can vary depending on the medium it is travelling through. It travels faster through solids than through liquids, and faster through gases than through solids. It also travels faster in humid conditions than in dry ones. Therefore, it is important to consider the environment when measuring the speed of sound.
In order to accurately measure the time it takes for sound to travel one mile, we need to use specialized tools such as an anemometer or ultrasonic sensor. An anemometer measures wind speed, while an ultrasonic sensor measures the time it takes for sound waves to travel from one point to another. Both of these tools can be used to measure the speed of sound over a mile.
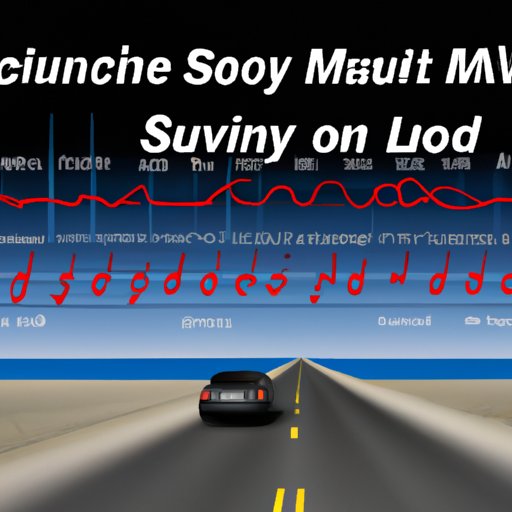
The Journey of Sound: Calculating How Long it Takes to Cover a Mile
Once we have the right tools, we can calculate how long it takes for sound to travel one mile. To do this, we first need to measure the speed of sound in the environment. We can then use this information to calculate the time it takes for sound to cover a certain distance. For example, if the speed of sound is 343 m/s and the distance is 1609.344 meters (1 mile), it would take 4.7 seconds for sound to reach one mile.
We can also use a digital sound level meter to measure the time it takes for sound to travel one mile. A sound level meter measures the intensity of sound in a given environment, allowing us to calculate the time it takes for sound to cover a certain distance. This can be useful for measuring sound in different environments, such as underwater or in a noisy city.
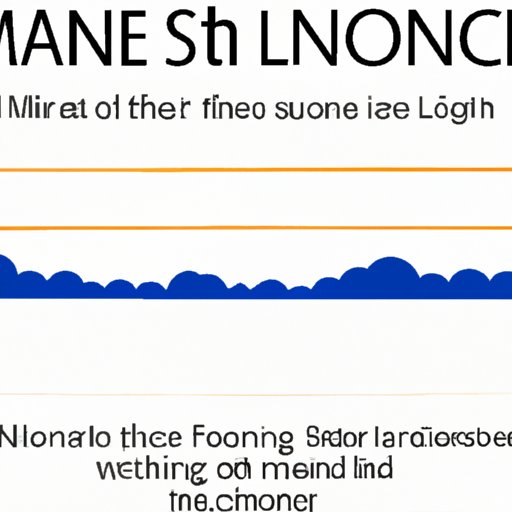
The Sounds of Distance: Investigating How Long it Takes for Sound to Reach One Mile
When measuring the speed of sound over a mile, there are several factors that can affect the results. These include the type of medium the sound is travelling through, the temperature of the environment, and the humidity levels. All of these factors can have an impact on the speed of sound, making it difficult to accurately measure how long it takes for sound to travel one mile.
It is also important to consider the type of environment in which the sound is being measured. Different environments can have different acoustic properties, which can affect the speed of sound. For example, sound travels faster in open spaces than in enclosed spaces, and faster in water than in air. Therefore, it is important to take into account the environment when measuring the speed of sound.
Timing is Everything: Determining How Long it Takes for Sound to Travel 1 Mile
Measuring the time it takes for sound to travel one mile requires patience and precision. It is important to use the right tools and take into account all of the factors that can affect the speed of sound. By doing so, we can accurately measure the time it takes for sound to reach one mile.
Once we have the data, we can analyze the results to determine the exact time it takes for sound to travel one mile. This information can be used to understand the speed of sound in different environments and to make predictions about how sound will travel in the future.
The speed of sound can vary depending on the environment, making it difficult to determine exactly how long it takes for sound to travel one mile. However, by taking into account the type of medium, the temperature, and the humidity of the environment, we can accurately measure the time it takes for sound to reach one mile. This information can be used to understand the speed of sound in different environments and to make predictions about how sound will travel in the future.
Understanding the speed of sound is essential for many applications, from medical imaging to audio engineering. By understanding how long it takes for sound to travel one mile, we can gain a better understanding of the power of sound and its potential to be harnessed and utilized.
(Note: Is this article not meeting your expectations? Do you have knowledge or insights to share? Unlock new opportunities and expand your reach by joining our authors team. Click Registration to join us and share your expertise with our readers.)
Hi, I'm Happy Sharer and I love sharing interesting and useful knowledge with others. I have a passion for learning and enjoy explaining complex concepts in a simple way.

Related Post
Exploring japan: a comprehensive guide for your memorable journey, your ultimate guide to packing for a perfect trip to hawaii, the ultimate packing checklist: essentials for a week-long work trip, leave a reply cancel reply.
Your email address will not be published. Required fields are marked *
Expert Guide: Removing Gel Nail Polish at Home Safely
Trading crypto in bull and bear markets: a comprehensive examination of the differences, making croatia travel arrangements, make their day extra special: celebrate with a customized cake.

Speed of sound to Miles per hour (mph)

Here you can convert the Speed unit Speed of sound into the unit Miles per hour and vice versa you can convert Miles per hour into Speed of sound. By clicking the "Swap units" icon, you will always obtain the desired conversion in the calculation result. With the following calculator you can also calculate any other Speed unit.
Info about "Speed of sound"
Definition: If an object travels at the speed of sound in air at 20 degrees Celsius for one second, it travels a distance of 343 meters per second. Actually, it is equivalent to 1.234,8 km/h.
The speed of sound is often illustrated by an example of a thunderstorm: if lightning strikes within 3 seconds of the thunder sound, then the thunderstorm is about 1 kilometer away. Another example, in a 100-meter sprint, the starting shot is fired directly next to the starting blocks. Imagine, if the start shot was fired at the finish line, it would take about 0.3 seconds of time to hear the shot in the start blocks.
Info about "Miles per hour"
The unit of length known as a "mile" and it should not be confused with the nautical mile and very commonly used in the Anglo-American countries. Therefore, the metric system is defined in miles (instead of kilometers) per time unit. For example, if a vehicle travels at a speed of 100 miles per hour (100 mph), it will move around 160 km per hour in the metric system.
The units are defined as follows: An object moving at 1 "mph" per hour will cover a distance of one mile. Therefore, 1 mile is equivalent to 1.609344 kilometers.
Formula for the conversion of Speed of sound to Miles per hour (mph) and vice versa
The calculation from Speed of sound to Miles per hour shall be made using the following conversion formula:
Formula for the conversion of Miles per hour (mph) to Speed of sound
The calculation from Miles per hour to Speed of sound shall be made using the following conversion formula:
Overview table : How many Speed of sound are in a Miles per hour ?
Overview table : how many miles per hour are in a speed of sound , what other readers have also read, source information.
As source for the information in the 'Units of speed' category, we have used in particular:
Last update
This page of the 'Units of speed' category was last edited or reviewed by Stefan Banse on February 19, 2023. It corresponds to the current status.
Changes in this category "Units of speed"
Rate our article with just one click.
All of cycling information sources
Home » Travel » Quick Answer: How Long For Sound To Travel 1 Mile
Quick Answer: How Long For Sound To Travel 1 Mile
It takes sound about 5 seconds to travel one mile.
How long does it take sound to travel 1 mile?
As you can see, the speed of sound changes depending on the temperature and the humidity; but if you want a round number, then something like 350 meters per second and 1,200 feet per second are reasonable numbers to use. So sound travels 1 kilometer in roughly 3 seconds and 1 mile in roughly 5 seconds.
Does sound travel at 1 mile per second?
In air at a temperature of 70° F (22.2° C), sound travels at a speed of about 1,129 feet (344 meters) per second, which is roughly equivalent to one-fifth of a mile in one second, or one mile in five seconds (or one kilometer in about three seconds).
How long does it take for light to travel 1 mile?
How long does it take light to travel certain distances? Distance Time 1 foot 1 nanosecond 1 mile 5.3 microseconds From New York City to Los Angeles 0.016 seconds Around Earth’s equator 0.133 seconds.
How fast is sound in miles per hour?
On Earth, the speed of sound at sea level — assuming an air temperature of 59 degrees Fahrenheit (15 degrees Celsius) — is 761.2 mph (1,225 km/h).
How fast does sound travel?
Sound can travel in air at approximately 332 metres per second. This is fast but not nearly as fast as light which travels at 300 000 kilometres per second. This difference in speeds enables us to appreciate that sound does take time to travel.
How many mph is Mach?
1 Mach (M) = 761.2 miles per hour (mph).
How far can sound waves travel?
The speed of the sound wave is 340 m/s. The distance can be found using d = v • t resulting in an answer of 25.5 m.
How far does sound travel per millisecond?
For reference, sound travels 0.343 meters per millisecond, so 10ms latency is the equivalent of sitting 3.4 meters from a speaker and playing your keyboard.
How far away is thunder when you hear it?
Thunder can be heard up to 25 miles away, and lightning strikes have been documented to occur as far as 25 miles from thunderstorms – known as a “bolt from the blue.” So if you can hear thunder, you’re close enough to be hit by lightning, and sheltering indoors or in an enclosed car is your safest bet.
Can humans travel at the speed of light?
So will it ever be possible for us to travel at light speed? Based on our current understanding of physics and the limits of the natural world, the answer, sadly, is no. So, light-speed travel and faster-than-light travel are physical impossibilities, especially for anything with mass, such as spacecraft and humans.
Is time Travelling possible?
In Summary: Yes, time travel is indeed a real thing. But it’s not quite what you’ve probably seen in the movies. Under certain conditions, it is possible to experience time passing at a different rate than 1 second per second.
How long would it take to travel 300 million miles?
The cruise phase begins after the spacecraft separates from the rocket, soon after launch. The spacecraft departs Earth at a speed of about 24,600 mph (about 39,600 kph). The trip to Mars will take about seven months and about 300 million miles (480 million kilometers).
How fast is a Mach 3?
The Mach 3 aircraft would be able to carry between nine and 19 passengers and fly at an altitude above 60,000 feet (18,300 metres). Featuring triangular delta wings, the aircraft would be capable of travelling at Mach 3, or 3704 kilometres per hour, which is three times the speed of sound.
What Mach breaks the sound barrier?
The moment an aircraft’s speed exceeds the speed of sound, it is said to have broken the sound barrier. At what speed do you break the sound barrier? The speed at which you break the sound barrier depends on many conditions, including weather and altitude. It’s approximately 770 mph or 1,239 kmh at sea level.
Does Mach 1 have fast?
By definition, at Mach 1, the local flow velocity u is equal to the speed of sound. At Mach 0.65, u is 65% of the speed of sound (subsonic), and, at Mach 1.35, u is 35% faster than the speed of sound (supersonic).
Does sound travel faster in cold air?
On a cold day, there tends to be a layer of warmer air above the cold pockets closest to the ground. Because sound moves faster in warm air than colder air, the wave bends away from the warm air and back toward the ground. That’s why sound is able to travel farther in chilly weather.
Does sound travel faster in denser air?
Just as solid objects allow sound to travel faster than less dense ones, the density of gasses affect how quickly sound travels, as well. You’d expect sound to travel faster in colder air than hotter air, because colder air is denser.
What does sound travel the fastest through?
Sound waves can be described by the wavelength and frequency of the waves. Sound travels more quickly through solids than through liquids and gases because the molecules of a solid are closer together and, therefore, can transmit the vibrations (energy) faster.
What Mach is the speed of lightning?
Around 13,000 ft (4,000 m) the Lightning would reach Mach 0.87 (1,009 km/h) and maintain this speed until reaching the tropopause, 36,000 ft (11,000 m) on a standard day.
What speed is mach5?
For objects traveling in dry air of a temperature of 20 °C (68 °F) at sea level, this speed is approximately 343.2 m/s (1,126 ft/s; 768 mph; 667.1 kn; 1,236 km/h). Speeds greater than five times the speed of sound (Mach 5) are often referred to as hypersonic.
How fast is light miles?
Light from a stationary source travels at 300,000 km/sec (186,000 miles/sec).
Related Posts
- How Does Sound Travel Per Mile
- Question: How Much Travel Expenses Per Mile
- Quick Answer: How Long To Travel Per Mile Dnd
- Question: How Much Is Travel Pay Per Mile
- Quick Answer: How Much Is Travel Reimbursement Per Mile
- How Much Per Mile For Travel Expenses
- How Many Points Per Mile Southwest
- Quick Answer: What Is Travel Rate Per Mile
- Question: How Much Companies Pay Per Mile For Travel
- Question: How Much To Charge Per Mile For Travel
- Quick Answer: What Can Travel A Mile Per Second
- Quick Answer: How Long Does A City Bus Travel Per Mile
- Quick Answer: How Long Does A City Bys Travel Per Mile
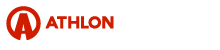
- Semi-Automatic Handguns
- Semi-Automatic Rifles
- Lever Action
- Bolt Action
- Handgun Build
- Rifle Build
- Shotgun Build
- Specialty Guns
- Handgun Parts
- Rifle Parts
- Thermal Vision
- Lasers & Lights
- Suppressors
- Shooting Rests
- Maintenance & Tools
- Rimfire Ammo
- Night Vision
- Fixed Blade
- Folding Knives
- Tactical Knives
- Less Lethal
- Flashlights
- Electronics
- Concealed Carry
- Self-Defense
- Hand to Hand Combat
- Home Defense
- Competition Shooting
- Precision Shooting/Long Range Shooting
- Shooting 101
- Tactical & LE
- Military Lifestyle
- New Products & Industry News
- Gun Facts & Laws
- Police News
- Military News
- Custom Black Rifle Build Off
- Free Gun Friday: Enter to Win the Kimber KDS9c
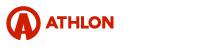
Long-Range Shooting: Basics of the One-Mile Shot

Twenty years ago or so, the idea of hitting a man-sized target at a mile (1,760 yards) was the stuff of legend. Yes, there were historical precedents, such as Union sniper Captain John T. Metcalf, US Army Corps of Engineers, who in 1864, reputedly shot a Confederate officer at a range of 1,666 meters (previously measured with a surveyor’s transit) using a heavy .50-caliber scoped muzzleloading rifle, and Carlos Hathcock’s 2,000-yard plus kill during the Vietnam conflict with a single shot from a .50BMG M2, but these were considered rare events done by almost superhuman marksmen and perhaps assisted by a bit of luck. Today, however, guns, cartridges, scopes, and more have enabled consistently accurate long-range shooting at 1,500 yards and much more. In Iraq and Afghanistan, for example, American soldiers with the .50BMG Barrett M107 have made more shots of that type than can be recorded.

Although the one-mile shot is no longer a once-in-a-lifetime event, it’s not something that one can do with a factory .30-06 hunting rifle and a 3-9x scope. Consistently hitting a man-size target at 1,700 yards or more requires a special combination of cartridge, rifle, optics and accessories.
Best Cartridges for Long-Range Shooting
Perhaps the single most important factor in achieving consistent long-range shooting accuracy is the selection of the proper cartridge. Generally, any cartridge for shooting at a mile or more should be capable of keeping its bullet above the speed of sound (around 1130 fps [feet per second] at 70 degrees Fahrenheit) at that distance. This, in turn, requires two things: a relatively high muzzle velocity of at least 2,800 to 2,900 fps, and a sharp-pointed, boattail match-type bullet having a high ballistic coefficient (BC).
Ballistic coefficient is a measure of how efficiently the bullet flies though the air. The higher the BC, the lower the drop, wind deflection and deceleration at any distance there is. High BC and high muzzle velocity combine to make range and windage estimation less critical. As a rule of thumb, any bullet for ultra-long-range shooting should have a BC of at least 0.650, and 0.700 to 0.800 (and higher) is better.
At present there are only a few production cartridges really suitable for military or law-enforcement shooting at 1,700 to 2,000 yards: the .338 Lapua, .338 Xtreme Tactical, .375 and .408 CheyTac, .416 Barrett and the .50BMG. There are also some wildcat, proprietary, or limited-production cartridges that may be suitable. The cartridges I listed, however, are widely available, factory pressure-tested with proven ballistic performance.
No 6.5mm, 7mm or .30-caliber magnums are included on my rather short list of one-mile sureshots; .338 is pretty much the minimum caliber for such work. There are two reasons for this. First, only a handful of bullets in these smaller diameters have the requisite high BCs of 0.700 and above. For technical reasons, the larger the bullet diameter, the higher the BC that is realistically achievable in a practical bullet design. Second, tactical shooters want the bullet to do something useful when it hits, and relatively light, small-diameter bullets have limited capabilities in this area. Even the 240-grain .30 caliber Sierra with a BC of 0.711, at a muzzle velocity of 3,000 fps, has only 759 fpe (foot-pounds of energy) left at 1,760 yards, which is about as much as a stiff .357Mag load out of a handgun. While this may injure unarmored enemy personnel, it probably won’t reliably penetrate a flak jacket, car body or building wall. In comparison, the cartridges on my list have anywhere from 1,000 to 3,800 fpe of remaining energy at that distance.
Cartridges for the one-mile shot should also exhibit high ballistic uniformity. A variance in muzzle velocity of 35 fps (2,850 fps and 2,885 fps) with the 300-grain .338 Sierra MatchKing yields a difference in drop of nearly a yard at a mile, enough to undershoot or overshoot an enemy sniper in a window. Velocity variations in the teens or single digits are best.
Best Rifles for Long-Range Shooting
Accuracy is clearly the prime requirement for any rifle to be used for ultra-long-range shooting. A man-size target 18 inches wide, at one mile is almost exactly 1-MOA (minute of angle) in size, so 1-MOA is the minimum precision required. Actually, given the variables of shooter skill, gun steadiness, wind, and so forth, a level of accuracy of 1/2- to 3/4-MOA at 1700 to 2000 yards is a more realistic minimum.
Though there are some self-loaders suitable for long-range shooting, bolt actions are generally preferred because of their inherent accuracy, strength, and reliability. The greater size of long-range rounds requires larger-than-normal actions, usually with diameters of 1.45 to 1.6 inches, and with larger bolts having beefier lugs. Barrels also tend to be hefty, as well as fairly long, 26 to 30 inches, to take full advantage of the heavy powder charges used in long-range cartridges. Muzzle brakes are common to cut recoil and allow lower gun weight. Suppressors are also widely used; though they don’t eliminate the supersonic crack of the bullet, they do reduce ground signature and muffle the muzzle blast, preventing easy detection of the shooter’s position.
Stocks for ultra-long-range rifles are most commonly of synthetic materials (fiberglass, Kevlar, graphite, etc.) and, aluminum. These materials are durable and strong, and unlike wood, are unaffected by water, solvents, or changes in temperature or humidity. Increasingly in demand are modular aluminum stocks with interchangeable subunits, adjustments for height and pull length, multiple provisions for mounting lights, bipods/monopods, night vision devices, and other accessories and a folding buttstock option. Such stocks can be easily reconfigured to meet changing operational requirements.
There is actually a pretty good selection of rifles suitable for ultra-range work. In .338 Lapua Mag, models include the Accuracy International AWM, ArmaLite AR-30A1, Barrett M98B, Blaser R93 Tactical, Dakota Longbow T-76, Sako TRG-42, and Surgeon XL. The other major option in .338 caliber is the powerful .338 Xtreme Tactical, capable of 2000+yard accuracy.
A step up in bullet diameter are the Model 200 and 310 CheyTac rifles, in .375 and .408 CheyTac cartridges as well as the rifles, and then there’s the Barrett Model 99 in .416 Barrett. And in .50 BMG, there are quite a few choices for tactical shooters, including models from Accuracy International, Barrett (both semi-auto and bolt-action models), McMillan, Robar, Steyr and others.
Long-Range Optics
Optics for one-mile shooting must be clear with low distortion and lots of light transmission. Preferred are variable-power models having a maximum magnification of at least 14-16x, and better 20x; variables allow the option of lower scope magnifications to scan a broad area to engage closer-range targets, or to reduce the effects of mirage. Powers of 30-40x and higher are not as popular, as they often come at the cost of smaller exit pupil size, less eye relief, and a decreased range of reticle adjustment.
Ultra-long-range scopes must be exceptionally rugged to withstand the recoil of powerful cartridges, and also must have a wide range of windage and elevation adjustments (up to 100 minutes or better on some models) to accommodate bullet drop and wind deflection at ranges approaching 2000 yards. Note that just about all scopes on long-range rifles are mounted on Picatinny rails having a 20- to 40-minute forward angle. The range of reticle adjustment increases with main tube size, which is why 30mm or larger tubes are preferred. Most commonly, click values are either 1/4-MOA or 1/10-milliradian (mil), at one-mile, these values are equal to 4.4 and 6.3 inches of bullet impact per click, respectively.
Reticle design is critical. Thick crosshairs will often obscure the target and fine crosshairs will be hard to see under reduced-light conditions. Almost all scopes for long-range work have reticles with some sort of rangefinding and/or drop compensation capability. In some scopes, this takes the form of circles or spaced lines designed to encompass 18 inches at various ranges, as well as a series of horizontal lines representing the aiming points for different yardages. The most common rangefinding system is the mil-dot reticle, which features round (Army) or oval (Marine Corps) dots that subtend either 0.20- or 0.25-mil and are spaced one-mil apart. Using simple mathematical formulas, or a device such as the Mil-Dot Master, such reticles permit fairly precise range and holdover/holdoff calculations. Scopes with mil-dot reticles are used by the US Marine Corps, US Army and other military organizations.
Reticles are placed in either the first or second focal plane. In the first focal plane, the reticle grows larger as the scope magnification is increased, while in second-focal-plane designs (what most Americans are used to), the reticle stays the same apparent size throughout the magnification range. With second-focal-plane scopes having range-finding reticles, such as the mil-dot design, the scope must be dialed to a specific magnification for the reticle to give accurate readings. On the other hand, a mil-dot reticle on a first-focal-plane scope can be used at any magnification, though the increasing reticle size may obscure part of the target at high magnification.
Although some contemporary “tactical” scopes feature mil-dot reticles and 1/4-MOA adjustments, many real-world professional feel that a mil-dot reticle is best used with mil click adjustments.
Only a few scope brands are found with any regularity on real-world long-range rifles here and abroad: Horus, IOR Valdada, Kahles, Leupold Mark 4, Nightforce NXS, Schmidt & Bender, US Optics and Zeiss.
Accessories
A shooter armed with a scoped rifle can make the one-mile shot, better ultra-long-range performance can be achieved with the proper shooting accessories.
Precise determination of the distance to the target is critical to accuracy, as an error in range estimation of only 25 yards at one mile can mean a difference in vertical impact of around 3 to 4 feet, more than enough to miss a target. While rangefinding reticles can get a shooter in the general ballpark, laser rangefinders give a precise measurement (plus or minus 1 yard) to the target. Models that can range out to 1,500 yards include the ATN Ranger as well as numerous models from Bushnell, Leupold and Newcon. For ranging to 2,000 and beyond, Newcon is pretty much it with four models for military, marine, surveying and other purposes.
Some laser rangefinder models also include an inclinometer, compass, separate operating modes for different operating conditions and the ability to measure target speed. Note that laser rangefinders often don’t work well when the target is small or low in contrast with the background, or when there is fog or rain.
Even when the exact range to the target is known, a variety of atmospheric factors can greatly influence bullet trajectory. Precise knowledge of such conditions can be achieved using portable weather stations. The simplest of these measure wind speed and temperature only while more sophisticated instruments, such as the top-of-the-line Kestrel 4500 indicate wind speed, temperature, pressure, humidity, time/date, altitude, and the angle of the prevailing wind to the target, which can calculate the effective wind value. However, since these devices only register conditions at the firing point, they don’t reveal if there are different conditions further downrange, almost a certainty when the target is a mile or more away.
Tactical shooters often fire from the top of a building or the side of a hill or mountain. Even relatively small angles up or down can require sizable trajectory adjustments at one mile. Inclinometers, such as the Angle Cosine Indicator from Sniper Tools Design Company LLC, are useful devices for such shooting, as they attach to the rifle and give the shooting angle of the rifle. More importantly, the ACI also gives the proper adjustment factor that, when multiplied by the straight-line range to the target, gives the effective range for proper holdover estimations. Some of the better laser rangefinders also have an inclinometer feature.
Proper rifle support is a must. When shooting off sandbags is not possible, bipods are universally employed. There are a number of good bipods like Versa-Pod and more, but the most common seems to be the ubiquitous Harris design, which has stood the tests of both time and extreme conditions.
Rifle cant is an often undiagnosed cause of lateral dispersion at extended range. As little as one-degree, an angle many shooters find difficult to discern, can produce 0.5 of an inch of dispersion at 100 yards. A number of devices are available to combat this problem, ranging from simple bubble levels that mount on the scope or Picatinny rail, to electronic devices such as the Deros Level Grouse that use lights to indicate when the rifle is not vertical. Devices such as the Level Grouse can be perceived in the peripheral vision while aiming through a scope, and thus have advantages over bubble levels, which require a shift in visual focus from the crosshairs.
Finally, precision shooters are increasingly coming to rely upon ballistic calculators. These devices allow the inputting or selection of a wide range of data, such as cartridge, bullet velocity and BC, target distance, atmospheric conditions, altitude and shooting angle, and so forth, which are used to yield trajectory information via an interface with preprogrammed ballistic software. Though they all function in unique ways, these ballistic calculators have the same purpose of enabling the shooter to confidently fire with the expectation of a first-round hit.
Final Notes
That about covers the basics of the equipment needed for making successful shots at a mile or more. That’s not the whole story, though. Most important of all is skill. Even with a 1/2-MOA rifle, high-magnification optics, laser rangefinder, ballistic calculator and more, a precision rifleman still needs superior shooting technique to achieve consistent hits at one mile or more. Moreover, technological gizmos can break if dropped or when subjected to adverse conditions; their batteries can go dead; and sometimes they just don’t work properly.
The well-schooled long-range shooter not only knows how to shoot, but is also skilled at range estimation, wind reading, and proper trajectory compensation for different atmospheric conditions, shooting angles and target speeds. And such skill cannot be taught, it must be learned through extensive study and practice.
All that is perhaps why there is still, quite deservedly, a mystique about the one-mile shot

MOST POPULAR
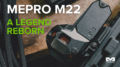
The New Mepro M22: The Legend Reborn
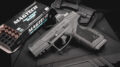
The Taurus GX4 Carry 9mm Is the Epitome of Reliability
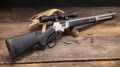
The New Smith & Wesson Model 1854 Lever-Action Rifle
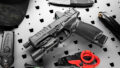
Springfield Armory Echelon 9mm

DESERT TACTICAL ARMS SRS COVERT 7.62mm

Hogue Unveils New G10 grips for the Ruger Mark IV

Gun Review: The 3-Inch-Barreled Ruger LCRx .38 Special Revolver
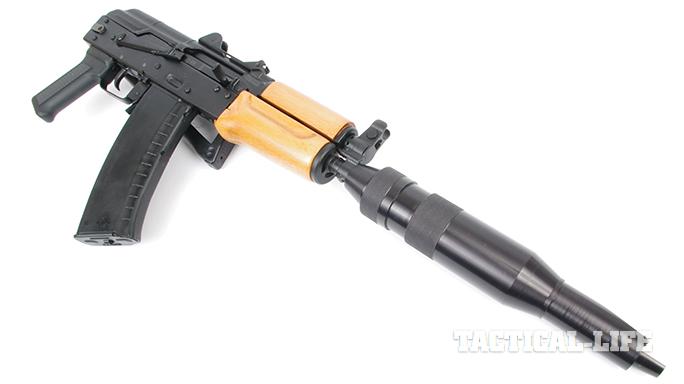
High Powered Armory’s Fake ‘Krink’ Suppressor
Browse by brand.
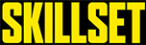
Springfield Armory SA-16A2 is a Must Have Retro Service Rifle
One could argue that the M16A2 was the rifle that made the civilian market for AR platform rifles in the...
VIDEO SERIES: AT THE READY | SEASON 2
More videos.

The Streamlight TLR RM 2 Delivers High Lumens and Red or Green Laser Options
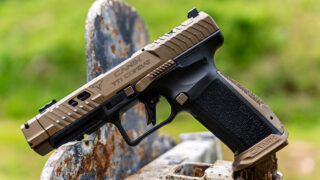
Finally! Thanks to CANiK, We Get an Affordable TTI Combat Pistol
Handguns - click to see all.

TESTED: Cabot Guns Enters the 2011 Market with its Insurrection

Little Dirty-S: Meridian Defense Corp’s All-American-Made AK

Survival Gear: Takedown Dark Mountain Arms Stowaway Series
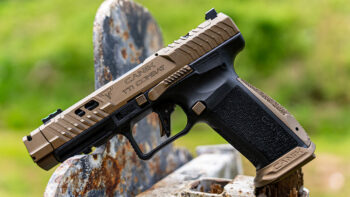
Meta Tactical Apex Conversion Kit: Pistol to Carbine in Minutes!
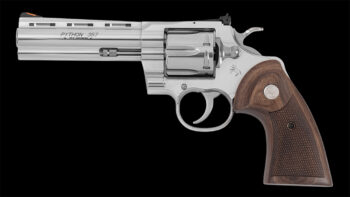
Colt Python Revolvers: 2.5 & 5-inch Stainless Steel Models Debut
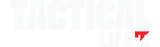
© 2023 Athlon Outdoors - Premium Firearm product and industry news, reviews and magazines" .
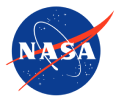
All Mars Resources
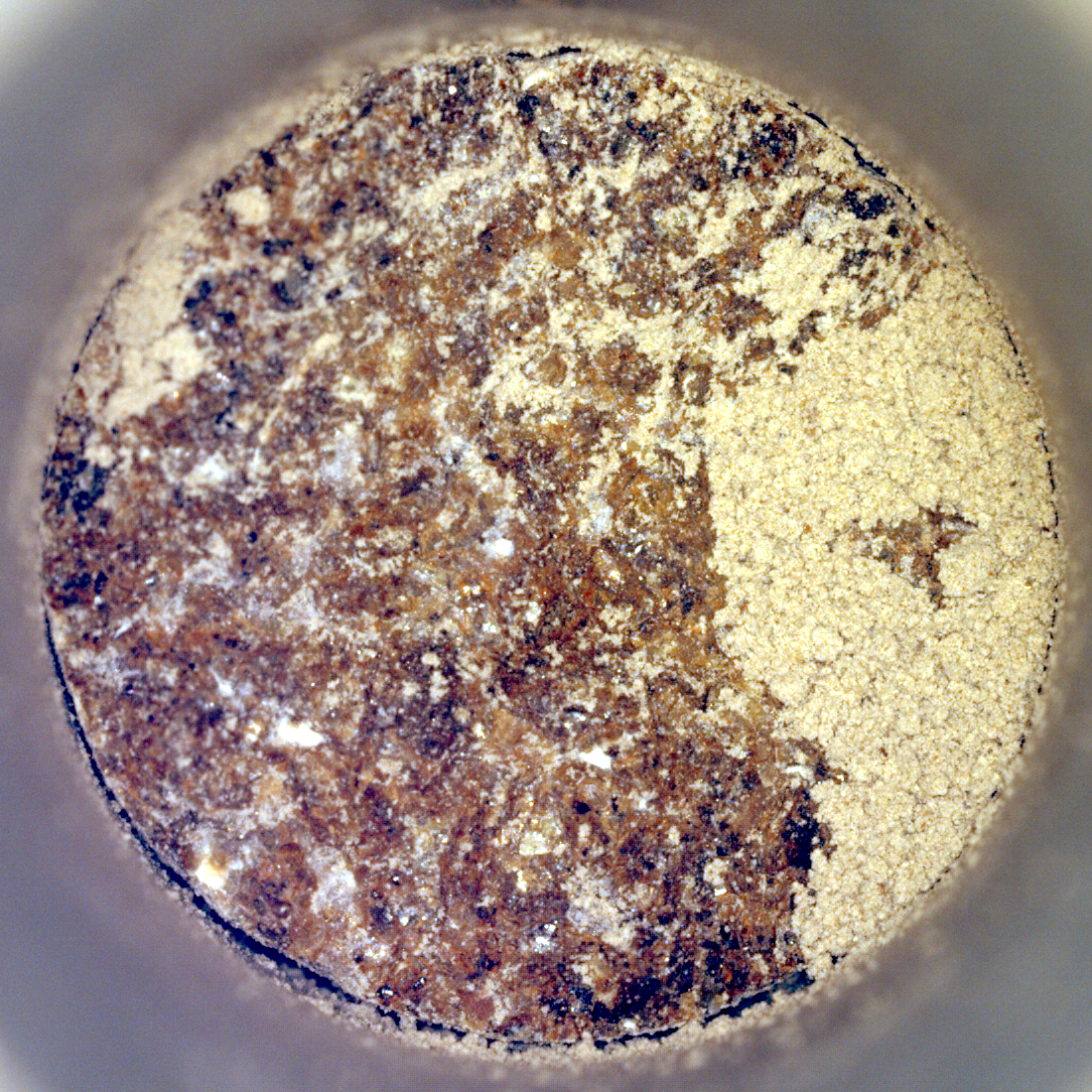
Perseverance’s ‘Bunsen Peak’ Sample
NASA’s Perseverance Mars rover captured this image of a sample cored from a rock called “Bunsen Peak” on March 11,…
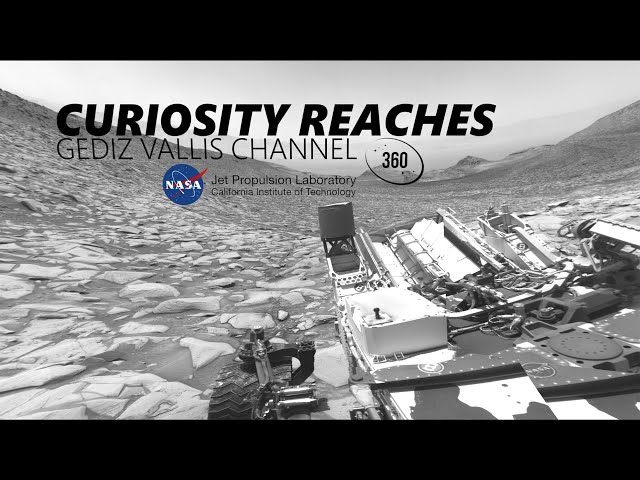
NASA’s Curiosity Rover Reaches Gediz Vallis Channel (360 View)
360-degree panorama provided by NASA’s Curiosity Mars rover. This view was captured at Gediz Vallis channel, a feature that formed…
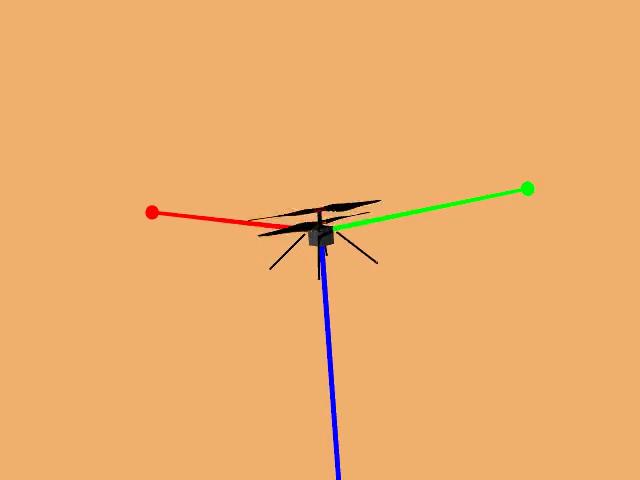
Animation of Mars Helicopter Flight Test
This animation shows a simulation of the response of NASA’s Ingenuity Mars Helicopter to the system identification, or “Sys-ID,” process.…
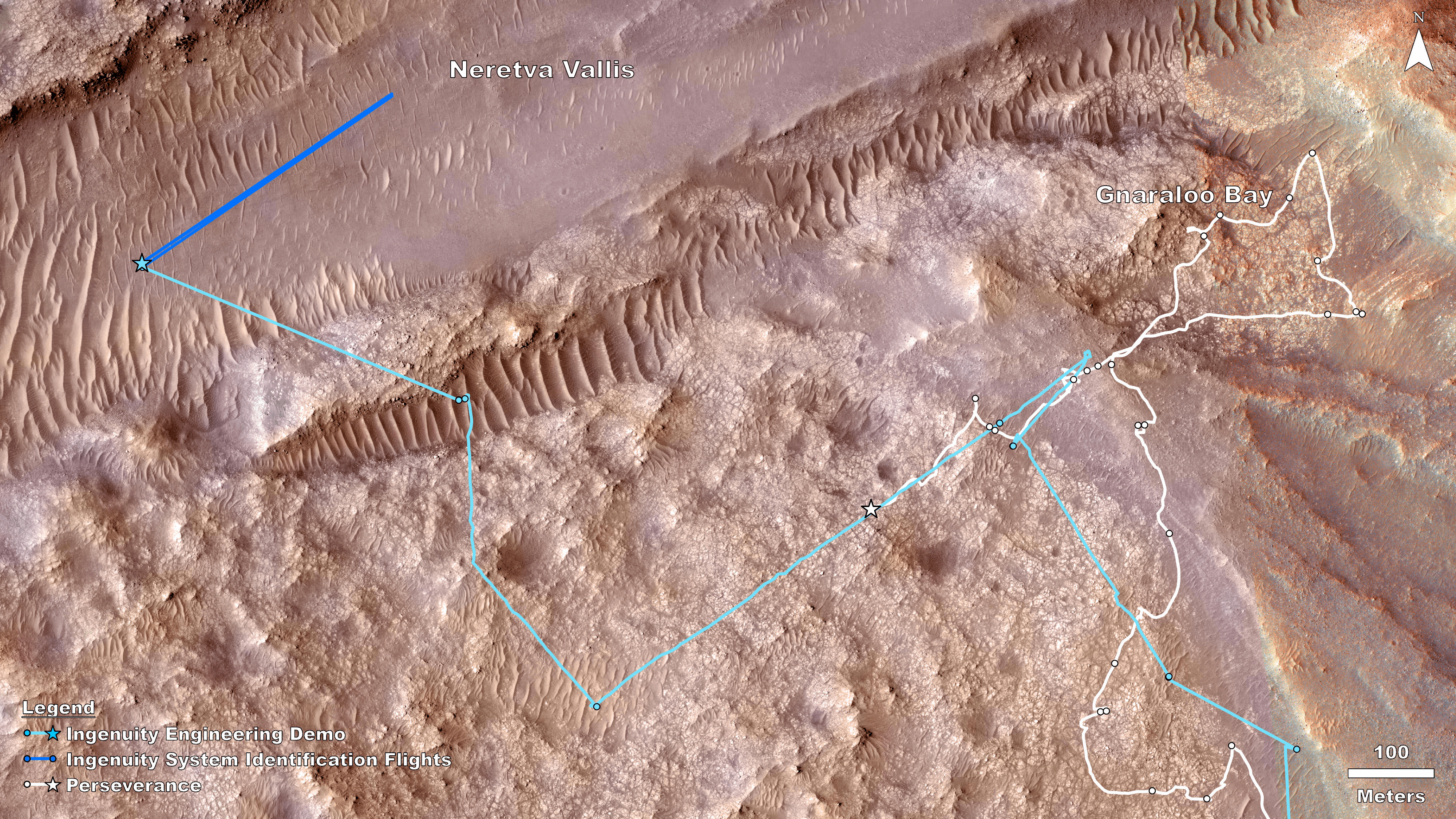
Rover, Helicopter Locations in Jezero Crater
This map shows the locations of NASA’ Perseverance rover (white star) and Ingenuity Mars Helicopter (cyan star) on Dec. 19,…
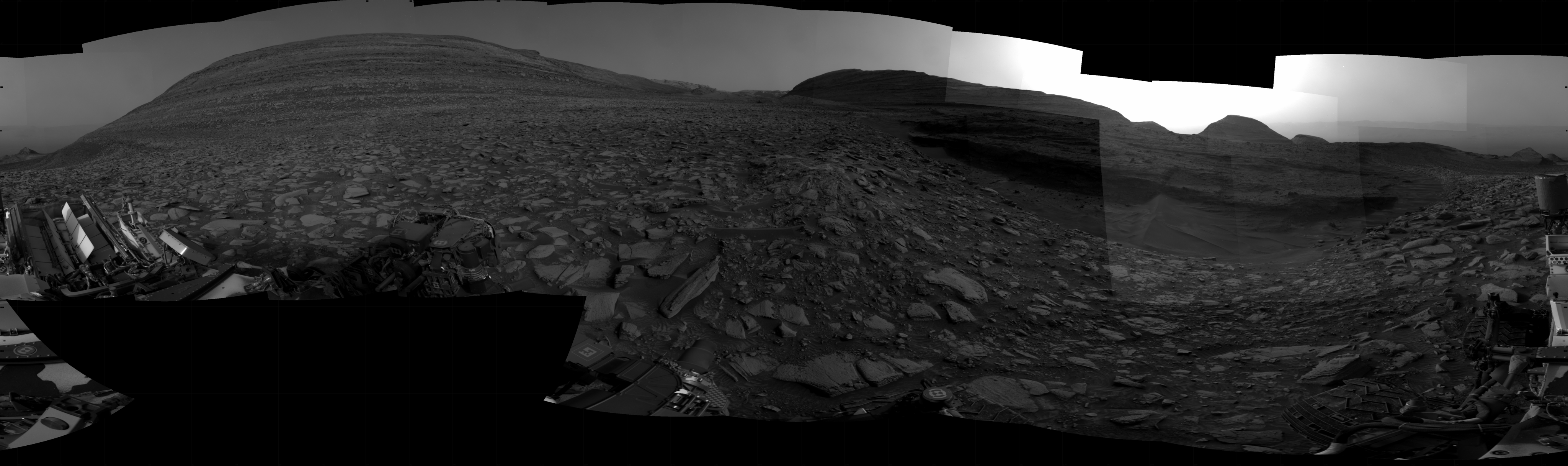
Sol 4132: Right Navigation Camera, Cylindrical Projection
NASA’s Mars rover Curiosity took 31 images in Gale Crater using its mast-mounted Right Navigation Camera (Navcam) to create this…
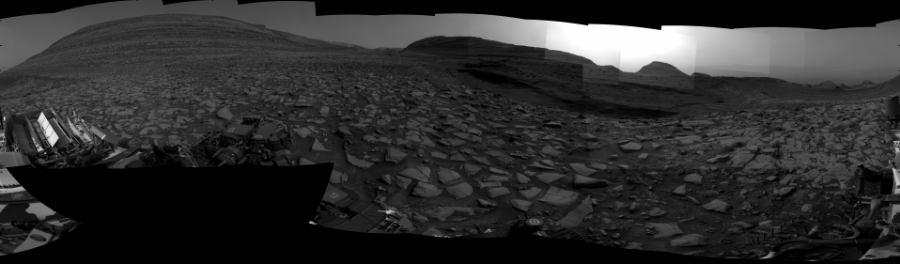
Sol 4130: Right Navigation Camera, Cylindrical Projection
NASA's Mars rover Curiosity took 31 images in Gale Crater using its mast-mounted Right Navigation Camera (Navcam) to create this…
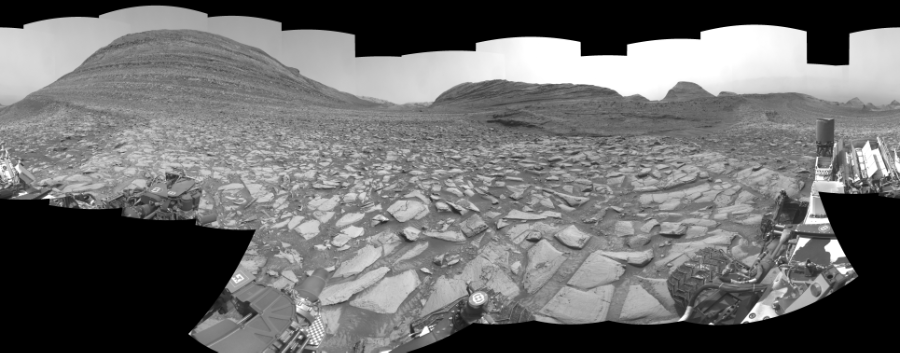
Sol 4128: Right Navigation Camera, Cylindrical Perspective
NASA's Mars rover Curiosity took 30 images in Gale Crater using its mast-mounted Right Navigation Camera (Navcam) to create this…
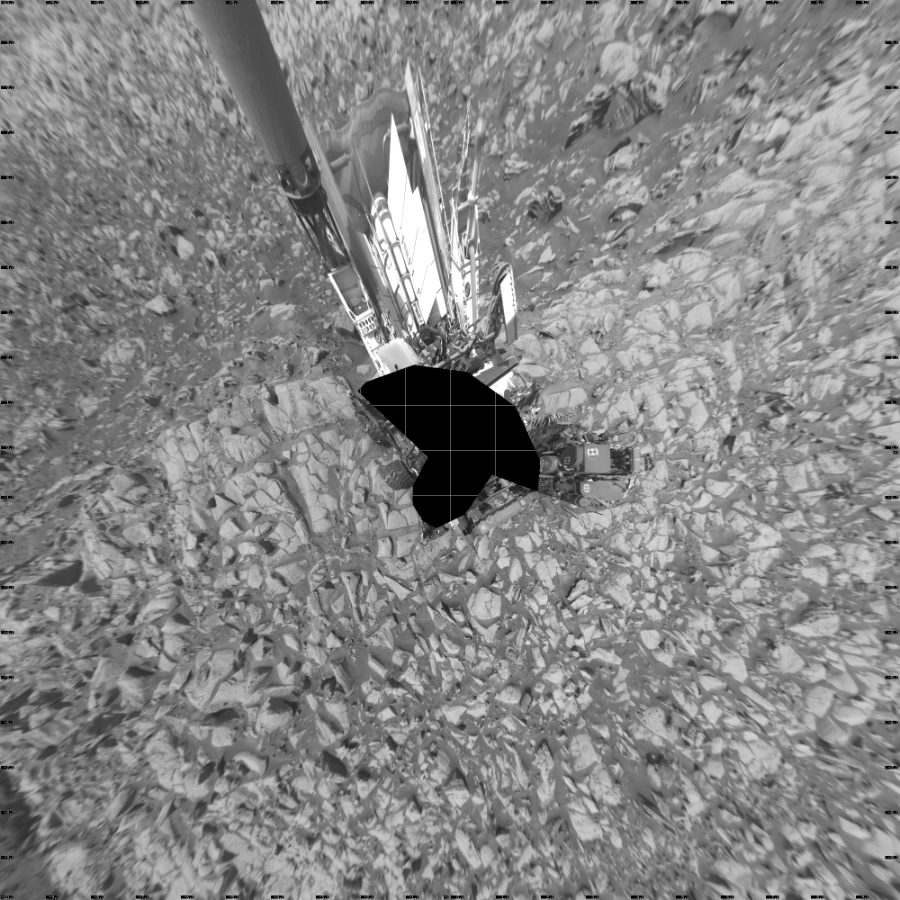
Sol 4128: Left Navigation Camera, Vertical Projection
NASA's Mars rover Curiosity took 30 images in Gale Crater using its mast-mounted Left Navigation Camera (Navcam) to create this…
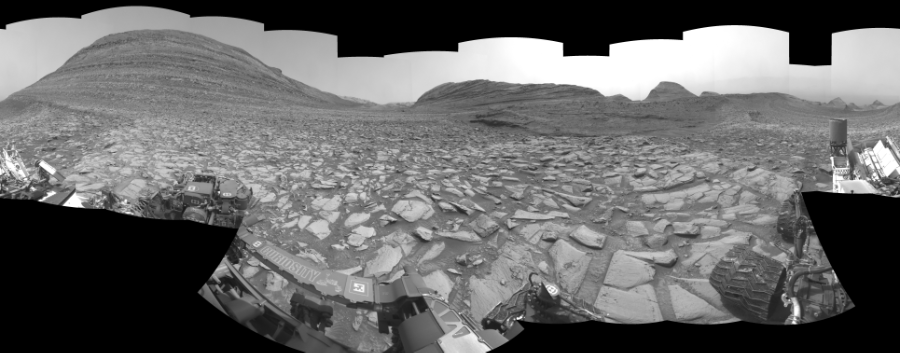
Sol 4128: Left Navigation Camera, Cylindrical Perspective
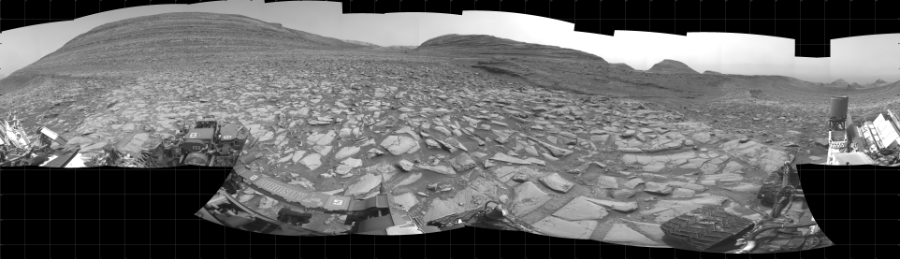
Sol 4128: Left Navigation Camera, Cylindrical Projection
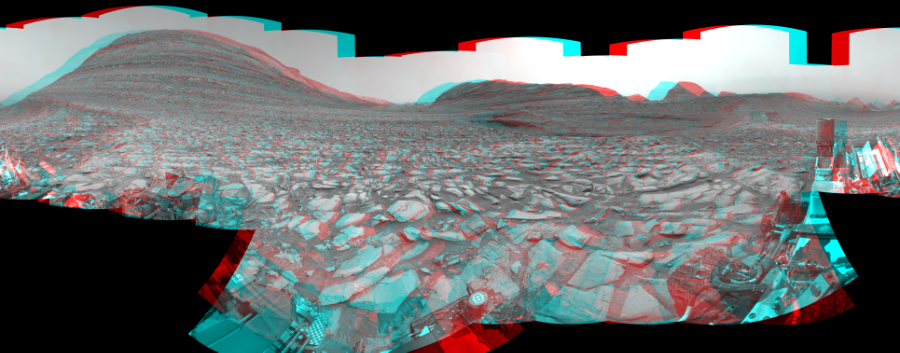
Sol 4128: Mast-Mounted Navigation Camera, Cylindrical Perspective
NASA's Mars rover Curiosity took 30 image pairs in Gale Crater using its mast-mounted Navigation Camera (Navcam) to create this…
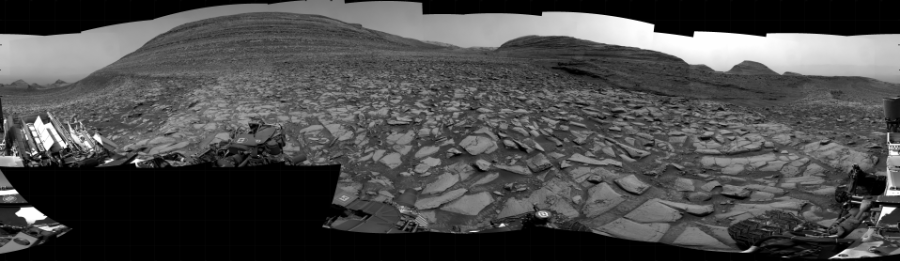
Sol 4128: Right Navigation Camera, Cylindrical Projection
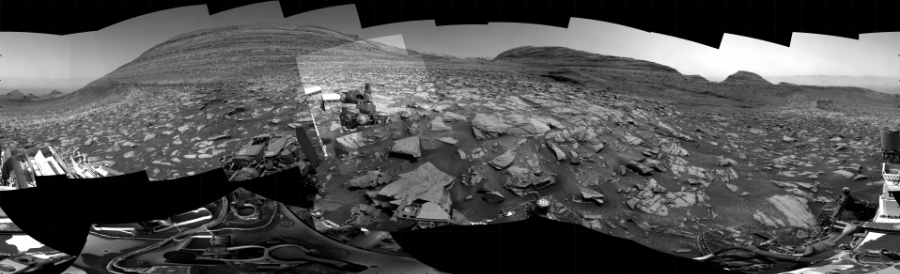
Sol 4125: Right Navigation Camera, Cylindrical Projection
NASA's Mars rover Curiosity took 52 images in Gale Crater using its mast-mounted Right Navigation Camera (Navcam) to create this…
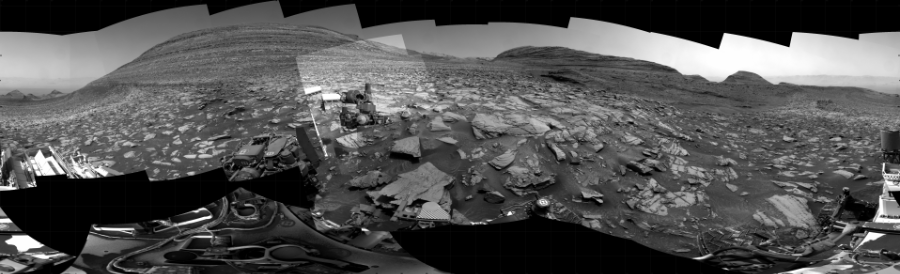
Sol 4123: Right Navigation Camera, Cylindrical Projection
NASA's Mars rover Curiosity took 51 images in Gale Crater using its mast-mounted Right Navigation Camera (Navcam) to create this…
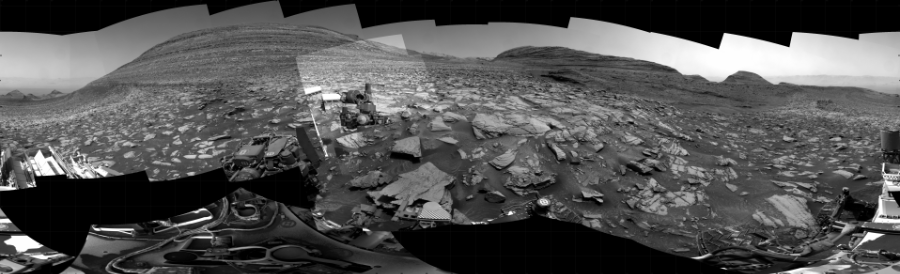
Sol 4118: Right Navigation Camera, Cylindrical Projection
NASA's Mars rover Curiosity took 49 images in Gale Crater using its mast-mounted Right Navigation Camera (Navcam) to create this…
Capital One miles: How to earn, redeem and transfer rewards

Transferable credit card rewards are especially valuable because of the many ways you can redeem them. You might be familiar with rewards like Chase Ultimate Rewards points and American Express Membership Rewards points. But, another transferable reward currency that can offer significant value to some travelers is Capital One miles .
Capital One miles are appealing for many reasons. For example, several Capital One cards that earn miles earn more than 1 mile per dollar spent on all purchases. And one Capital One card that earns miles only requires good credit. Plus, you should always be able to get at least 1 cent per mile when you redeem — and you may get significantly more value if you use Capital One's transfer partners .
So, here's everything you need to know about Capital One miles.
What are Capital One miles?
Capital One miles are the rewards you earn with select Capital One cards , including the Capital One Venture X Rewards Credit Card and Capital One Spark Miles for Business .
You can redeem these miles in many ways, including transferring to select airline and hotel loyalty programs, redeeming for travel book ed through the Capital One travel portal and redeeming to cover recent travel purchases.
Related: Who should (and shouldn't) get the Capital One Venture X card?
How to earn Capital One miles
You can earn Capital One miles through select credit cards. In particular, most Capital One credit cards offer an initial sign-up bonus when you open a new card and meet the specified spending requirements to earn the bonus. You'll also earn miles on most purchases, and some cards even offer anniversary bonus miles each year you renew your card.
Although Capital One offers many different credit cards, the Capital One cards that earn miles are those in the Venture and Spark Miles families. Here's a quick look at these cards and who they are best for:
- Capital One Venture X Rewards Credit Card : Best for earning Capital One miles if you'll use the $300 Capital One Travel credit each account anniversary year ($395 annual fee; see rates and fees )
- Capital One Venture Rewards Credit Card : Best for earning Capital One miles ($95 annual fee; see rates and fees )
- Capital One VentureOne Rewards Credit Card : Best for earning Capital One miles without paying an annual fee ($0 annual fee; see rates and fees )
- Capital One VentureOne Rewards for Good Credit : Best for earning Capital One miles with good credit ($0 annual fee; see rates and fees )
- Capital One Venture X Business : Best business card for earning Capital One miles if you'll use the $300 Capital One Travel credit each account anniversary year ($395 annual fee; see rates and fees )
- Capital One Spark Miles for Business : Best business card for earning Capital One miles with a sub-$100 annual fee ($0 introductory annual fee for the first year, $95 after that; see rates and fees )
- Capital One Spark Miles Select for Business : Best business card for earning Capital One miles without paying an annual fee ($0 annual fee)
The information for the Capital One Spark Miles Select card has been collected independently by The Points Guy. The card details on this page have not been reviewed or provided by the card issuer.
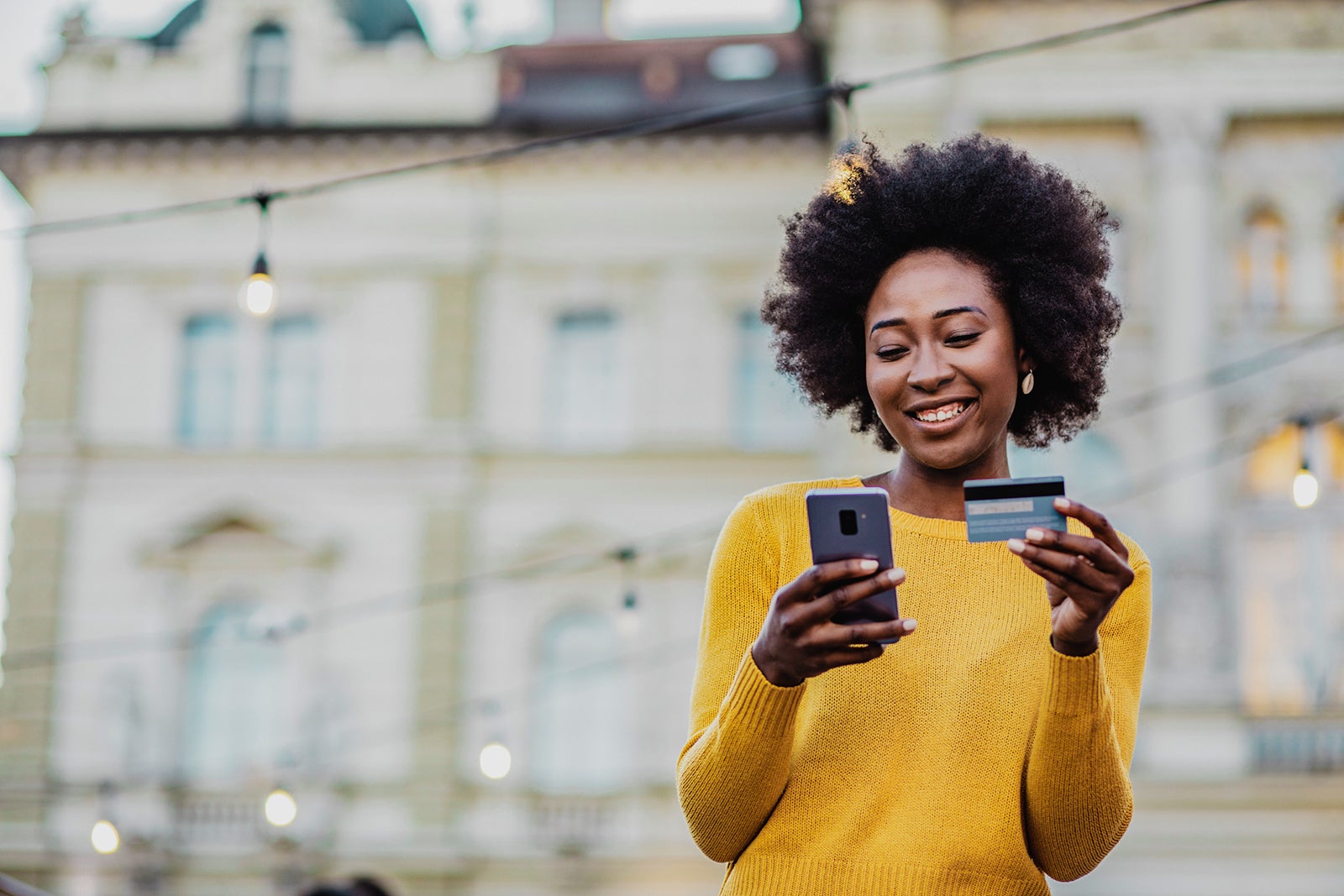
You can convert Capital One cash back into miles if you have one of the above cards. That means if you have a Capital One card like the Capital One SavorOne Cash Rewards Credit Card that earns cash-back rewards, you can convert your cash-back rewards into Capital One miles if you also have a card that earns Capital One miles.
I have the Capital One Venture X Rewards Credit Card and plan to keep it long-term since it offers perks that more than offset its $395 annual fee (see rates and fees ). After all, I easily use the $300 Capital One Travel credit each account anniversary year by booking flights through the travel portal, and the 10,000-mile anniversary bonus is worth about $185 based on TPG's valuations . But check out our Capital One Venture X card review to learn about its many other valuable perks.
Related: The best time to apply for these popular Capital One credit cards based on offer history
Capital One transfer partners
Capital One partners with several airline and hotel loyalty programs. You can transfer Capital One miles to these partner programs and then redeem through these loyalty programs if you wish.
Here's a look at the current Capital One transfer partners and the standard transfer ratios:
- Accor Live Limitless : 2:1 transfer ratio
- Aeromexico Rewards : 1:1 transfer ratio
- Air Canada Aeroplan : 1:1 transfer ratio
- Air France-KLM Flying Blue : 1:1 transfer ratio
- Avianca LifeMiles : 1:1 transfer ratio
- British Airways Executive Club : 1:1 transfer ratio
- Cathay Pacific Asia Miles : 1:1 transfer ratio
- Choice Privileges : 1:1 transfer ratio
- Emirates Skywards : 1:1 transfer ratio
- Etihad Airways Guest : 1:1 transfer ratio
- EVA Airways Infinity MileageLands : 4:3 transfer ratio
- Finnair Plus : 1:1 transfer ratio
- Qantas Frequent Flyer : 1:1 transfer ratio
- Singapore Airlines KrisFlyer : 1:1 transfer ratio
- TAP Air Portugal Miles&Go : 1:1 transfer ratio
- Turkish Airlines Miles&Smiles : 1:1 transfer ratio
- Virgin Red : 1:1 transfer ratio
- Wyndham Rewards : 1:1 transfer ratio
Transfer bonuses may occasionally let you transfer Capital One miles to one or more partner programs at an elevated rate. Remember, transfers are one-way: Transferring rewards back to Capital One miles is almost always impossible once you've transferred them to a loyalty program.
Related: Why transferable points are worth more than other rewards
How to redeem Capital One miles
You'll see multiple options for redeeming Capital One miles in your online account.
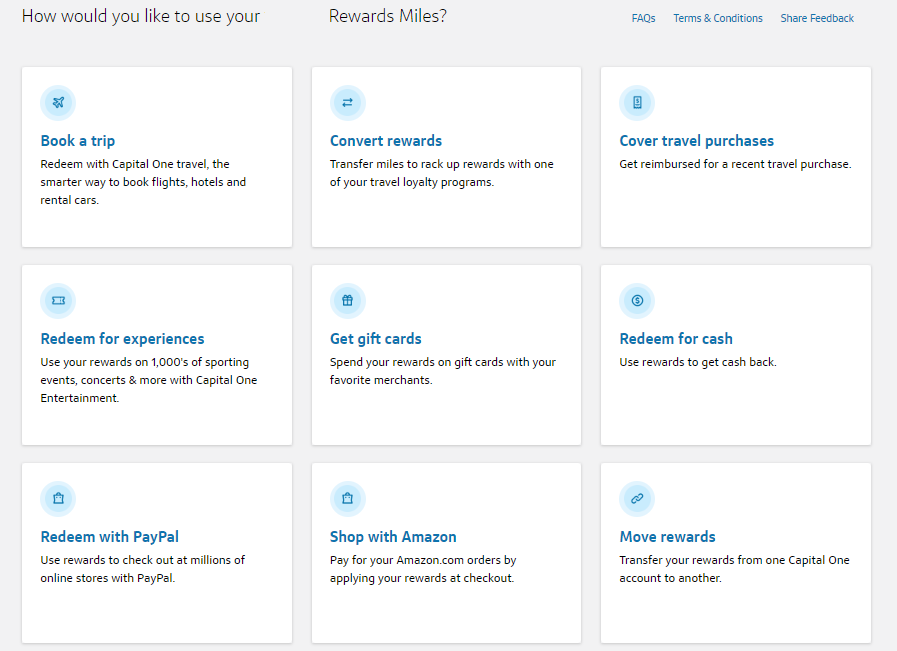
You'll typically get the best value when you transfer your miles to airline and hotel partners, but you can also get decent value when booking through the Capital One travel portal or redeeming miles to offset recent travel purchases. Here are your options and the redemption value each should provide.
Redeem with airline and hotel partners
As mentioned above, you'll typically get the best value from your miles when you transfer them to airline and hotel partners by clicking "Convert rewards." In the previous section, I listed the Capital One transfer partners and the ratios at which you can transfer Capital One miles to each partner.
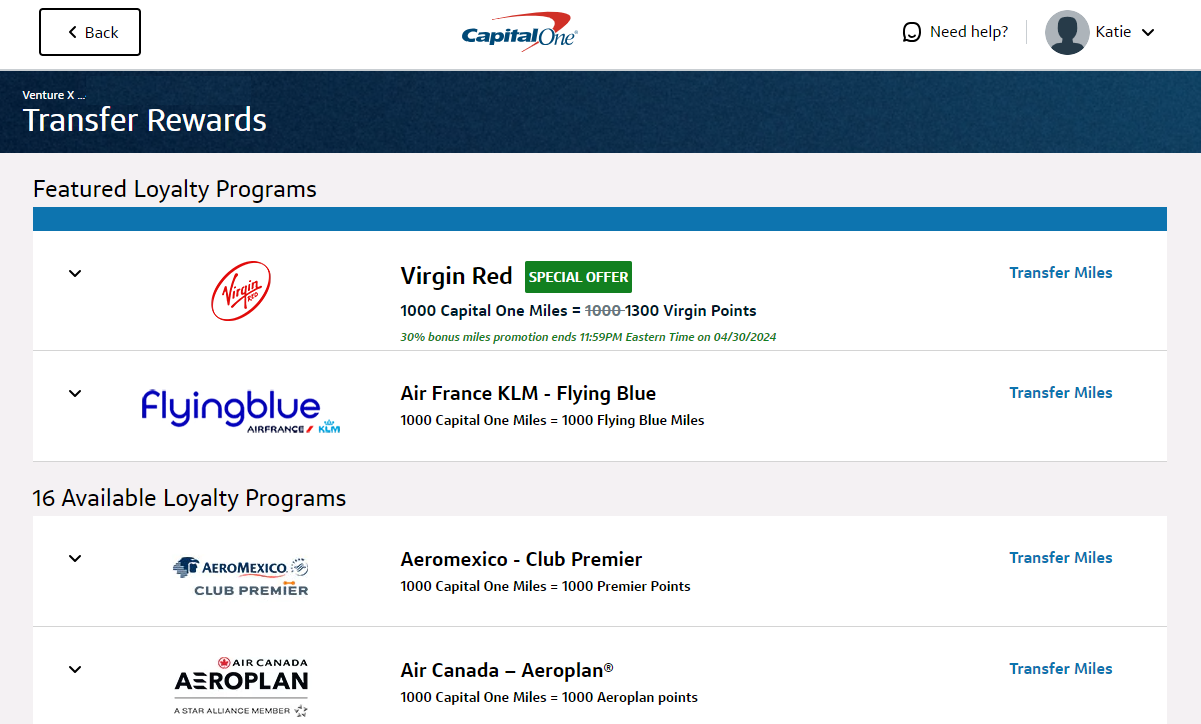
Based on TPG's valuations, you can expect to get about 1.85 cents per Capital One mile when you redeem with airline and hotel partners. However, the exact value you get will depend on which transfer partners you use and the types of awards you book with these partners. Check out our story on maximizing Capital One miles for some recommendations.
Related: How to transfer Capital One miles to airline and hotel partners
Book through the Capital One travel portal
You can also redeem miles at a rate of 1 cent per mile when booking flights, hotels and rental cars through the Capital One travel portal . However, if you book paid travel through the Capital One travel portal with your Capital One card that earns transferable miles, you can earn as follows:
- Venture X and Venture X Business : 10 miles per dollar on hotels and rental cars and 5 miles per dollar on flights booked through Capital One Travel
- Venture, VentureOne, VentureOne for Good Credit, Spark Miles and Spark Miles Select : 5 miles per dollar on hotels and rental cars booked through Capital One Travel
As such, I recommend booking paid rates instead of redeeming Capital One miles when you book travel through the Capital One travel portal. Even if you want to redeem miles for your flight, hotel or car rental booking, you'll often be better off using miles to cover recent travel purchases instead of redeeming when booking through the travel portal.
Related: Should you transfer Capital One miles to partners or redeem directly for travel?
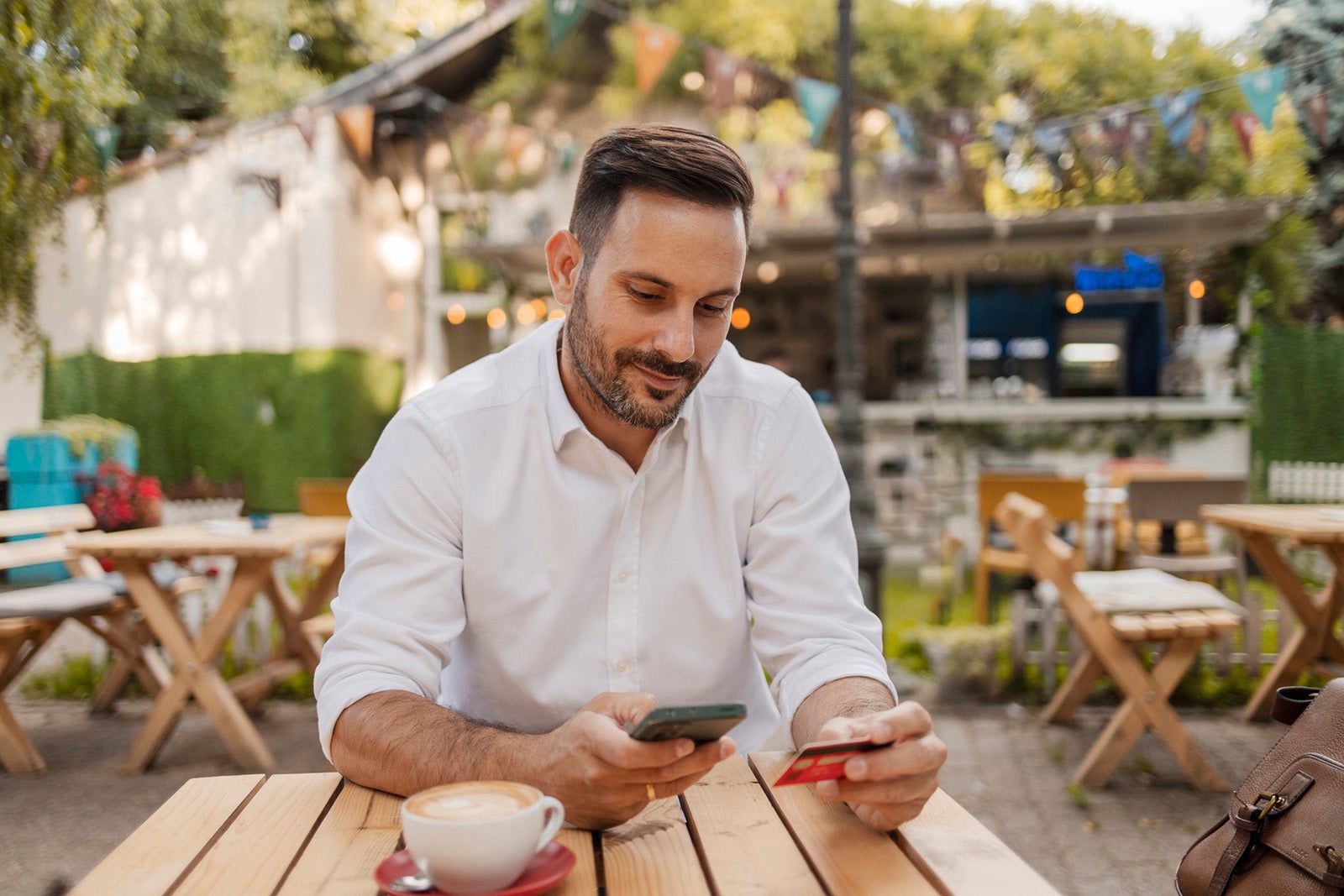
Cover travel purchases
You can redeem Capital One miles to cover recent travel purchases made with your card. Capital One says eligible travel purchases usually include those you make with "airlines, hotels, rail lines, car rental agencies, limousine services, bus lines, cruise lines, taxi cabs, travel agents and time shares." However, whether a specific purchase is eligible depends on the merchant category code attached to the purchase.
You'll see any eligible purchases — which are travel purchases posted to your account in the last 90 days — listed in your online account when you click "Cover travel purchases."
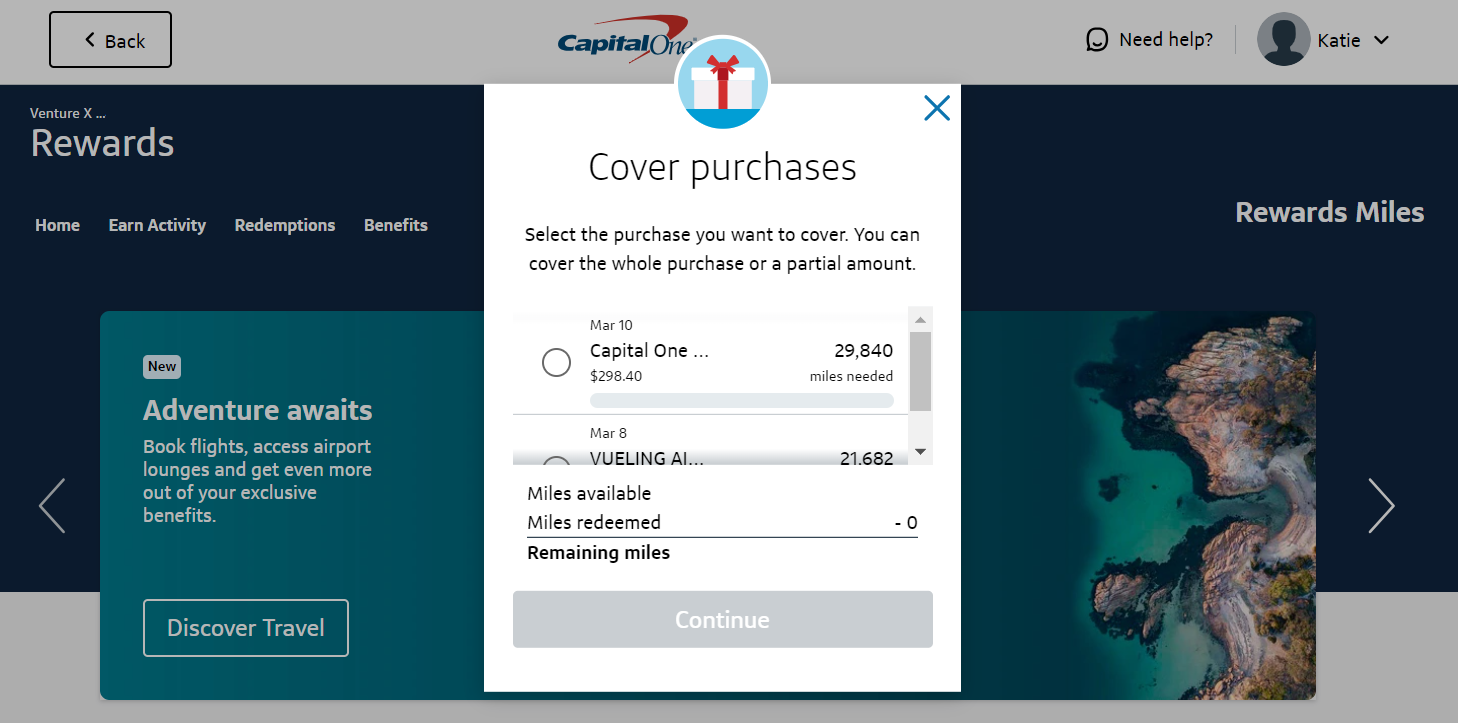
You can opt to cover the entire purchase if you have enough miles. Or, you can use miles to cover just part of the purchase. Either way, your miles are worth 1 cent each when you redeem them to cover recent travel purchases.
Related: How to redeem Capital One miles at a fixed value
Other ways to redeem Capital One miles
As you might have noticed in the screenshot above, cardholders have several other ways to redeem Capital One miles. Most of these other redemption options offer lower redemption values, so we don't generally recommend using them. But here's an overview of your other redemption options:
- Redeem for experiences : You can redeem miles for sports and concert tickets through Capital One Entertainment, usually at a redemption rate of 0.8 cents per mile.
- Get gift cards : You can redeem miles for gift cards, usually at a redemption rate of 0.8 to 1.067 cents per mile.
- Redeem for cash : You can request a statement credit or a check to your address at a redemption rate of 1 cent per mile.
- Redeem with PayPal : You can apply miles when checking out with PayPal at a redemption rate of 0.8 cents per mile.
- Shop with Amazon : You can apply miles at checkout for your Amazon.com orders at a redemption rate of 0.8 cents per mile.
As you can see, there are many ways to redeem Capital One miles for at least 1 cent each. So, there will rarely be a reason to redeem Capital One miles for anything less than 1 cent each.
Bottom line
When traveling overseas, I often use the Capital One Venture X Rewards Credit Card as my everyday spending card. It doesn't charge foreign transaction fees, I know I'll earn at least 2 miles per dollar on all purchases, and I rarely have issues using it. But, I also generally find it useful to have access to Capital One miles when booking award flights. So, I'm always happy to earn more Capital One miles on my everyday purchases.
Now that you know how to earn and redeem Capital One miles, I expect you may also understand their value — especially if you transfer your miles to airline or hotel partners.

IMAGES
VIDEO
COMMENTS
The speed of sound calculator displays the speed of sound in water; it's 4672 ft/s. Let's compare it with 90 °F (warm bath temperature). The speed is equal to 4960 ft/s this time. Remember that you can always change the units of speed of sound: mph, ft/s, m/s, km/h, even to knots if you wish to. ...
So sound travels 1 kilometer in roughly 3 seconds and 1 mile in roughly 5 seconds. Advertisement. When you see the flash of a lightning bolt, you can start counting seconds and then divide to see how far away the lightning struck. If it takes 10 seconds for the thunder to roll in, the lightning struck about 2 miles or 3 kilometers away. ...
As one particle is pushed, it compresses the neighboring particles, creating a chain reaction of compressions and rarefactions that travels through the air at a specific speed, approximately 767 miles per hour (1,235 kilometers per hour) at room temperature. ... Calculating Sound Travel Time in Air for 1 Mile: To understand the essence of sound ...
by Tim Brice and Todd Hall. The speed of sound depends on several variables, but the only independent variable we need to calculate the speed of sound is the temperature of the air. Enter your air temp and choose your units: The speed of sound: mph. Fahrenheit.
The speed of sound is the distance travelled per unit time by a sound wave as it propagates through an elastic medium. In dry air at 20 °C (68 °F), the speed of sound is 343.2 metres per second (1,126 ft/s; 1,236 km/h; 768 mph; 667 kn), or a kilometre in 2.914 s or a mile in 4.689 s.In common everyday speech, speed of sound refers to the speed of sound waves in air.
Calculation of sound travel time in one mile of water; Sound Travel Time in Different Media. Comparison of sound travel times in various media; Discussion on how media properties affect the speed of sound; X. Real-life Applications. Explanation of practical uses of understanding sound travel time; Examples in fields like underwater acoustics ...
In this article, we will explore the factors that affect the speed of sound and provide a detailed answer to the question of how long it takes for sound to travel 1 mile. The Speed of Sound. The speed of sound is defined as the distance that a sound wave travels per unit of time. In other words, it is the rate at which a sound wave propagates ...
The speed of sound is the distance travelled per unit of time by a sound wave as it propagates through an elastic medium. At 20 °C (68 °F), the speed of sound in air is about 343 m/s (1,125 ft/s; 1,235 km/h; 767 mph; 667 kn), or one km in 2.91 s or one mile in 4.69 s.It depends strongly on temperature as well as the medium through which a sound wave is propagating.
In non-humid air at 20 degrees Celsius, the speed of sound is about 343 meters per second or 767 miles per hour. We can also watch the speed of sound of a repeating simple harmonic wave. The speed of the wave can again be determined by the speed of the compressed regions as they travel through the medium.
At normal atmospheric pressure and a temperature of 20 degrees Celsius, a sound wave will travel at approximately 343 m/s; this is approximately equal to 750 miles/hour. While this speed may seem fast by human standards (the fastest humans can sprint at approximately 11 m/s and highway speeds are approximately 30 m/s), the speed of a sound wave ...
For example, if we want to know how far a sound will travel in one second, we have: distance = 343 m/s x 0.001 s = 343 m. So sound travels 1 kilometer in roughly 3 seconds and 1 mile in roughly 5 seconds. ... Can Sound Travel 20 Miles? The air may be permeable to these lower-frequency, sub-audible sound waves generated by elephants. ...
Light travels at 186,291 miles per second (~300,000 km/s), and the speed of sound in dry air is equal to 0.21-0.22 miles per second (0.33-0.35 km/s), as it varies with the temperature. We can notice the lightning almost immediately even though the storm is far from us, but the thunder needs around 5 seconds to travel one mile and approximately ...
Speed of Sound Calculator. Calculator for determining the time it takes for sound to travel a distance and for the distance traveled. Helpful, e.g. during a thunderstorm, where lightning can be seen first and then thunder can be heard a few seconds later. The speed of sound at 20°C and high humidity is approx. 343 m/s (= 1235 km/h), at cooler temperatures and low humidity it is a little bit ...
A sound's amplitude shrinks as it travels, but its speed remains constant. The basic equation for constant speed motion (shown below) applies to sound. d = vt d = v t. In this equation, d d represents the distance traveled by the sound, t t represents the amount of time it took to go that distance and v v represents the speed.
Thunder is the sound caused by a nearby flash of lightning and can be heard for a distance of only about 10 miles from the lightning strike. The sound of thunder should serve as a warning to anyone outside that they are within striking distance of the storm and need to get to a safe place immediately! Thunder is created when lightning passes ...
On a typical day at sea level sound travels at 761.1 mph. As our altitude increases there are changes in air density as well as temperature and the speed of sound also changes, see. Speed of Sound Table and Formula. Thus, the time required for sound to travel one mile is: time (seconds) = ( 761.1 mile/hr) * (1 hr / 3,600 sec) time = 0.2114 seconds
The fastest speed at which sound can travel in air, often referred to as Mach 1, is approximately 767 miles per hour (1,235 kilometers per hour) at sea level and 68°F (20°C). When an aircraft exceeds this speed, it pierces through the sound barrier, producing the iconic sonic boom, a testament to the remarkable physics of sound.
There is one crucially important difference between waves bumping over the sea and the sound waves that reach our ears. Sea waves travel as up-and-down vibrations: the water moves up and down (without really moving anywhere) as the energy in the wave travels forward. Waves like this are called transverse waves.
We will look at the different ways to measure sound over a mile, as well as calculations that can help us determine the exact time it takes for sound to reach one mile. Exploring the Speed of Sound: How Long Does it Take for Sound to Travel 1 Mile? The speed of sound is affected by various factors, including the type of medium it is travelling ...
Definition: If an object travels at the speed of sound in air at 20 degrees Celsius for one second, it travels a distance of 343 meters per second. Actually, it is equivalent to 1.234,8 km/h. ... The calculation from Speed of sound to Miles per hour shall be made using the following conversion formula: Conversion formula Speed of sound to Miles ...
Experts say the sound of thunder travels one mile every five seconds, so that can help you discern the distance of a lightning strike
So sound travels 1 kilometer in roughly 3 seconds and 1 mile in roughly 5 seconds. Does sound travel at 1 mile per second? In air at a temperature of 70° F (22.2° C), sound travels at a speed of about 1,129 feet (344 meters) per second, which is roughly equivalent to one-fifth of a mile in one second, or one mile in five seconds (or one ...
Accuracy is clearly the prime requirement for any rifle to be used for ultra-long-range shooting. A man-size target 18 inches wide, at one mile is almost exactly 1-MOA (minute of angle) in size, so 1-MOA is the minimum precision required. Actually, given the variables of shooter skill, gun steadiness, wind, and so forth, a level of accuracy of ...
The National Aeronautics and Space Administration. NASA explores the unknown in air and space, innovates for the benefit of humanity, and inspires the world through discovery. About NASA's Mission. Join Us. Home. News & Events. Multimedia. NASA+. Missions.
You can also redeem miles at a rate of 1 cent per mile when booking flights, hotels and rental cars through the Capital One travel portal. However, if you book paid travel through the Capital One travel portal with your Capital One card that earns transferable miles, you can earn as follows: Venture X and Venture X Business: 10 miles per dollar ...
One day after destructive tornadoes plowed through Nebraska and Iowa, millions of people in parts of Kansas, Missouri and Oklahoma were under tornado watches Saturday evening.