Ten Things to Consider When Comparing AC vs. DC Power Transmission
09-09-2019 | By Gary Elinoff
The story begins with a clash between Thomas Edison, the greatest inventor of all time, with Nicola Tesla, arguably the father of electrical engineering. Edison had demonstrated the practicality of his electric light-bulb, and in order for the world (or at least lower Manhattan) to enjoy the fruits of his work, a way to power it had to be standardized. Edison preferred DC , Tesla argued for AC.
The problem with DC was, at that time, it was impossible to efficiently convert high voltage DC to low voltage DC, or vice versa. AC could easily be converted, with the same type of magnetic transformers that are still so widely in use today.
The key to voltage conversions using an AC transformer is that power, defined as voltage-time current, is conserved. For example, 1,000 volts at 10 amps is 10,000 watts. If a transformer is used to step the voltage down to 100 volts, 100 amps will be available because 100 volts times 100 amps is 10,000 watts.

1- Why is Converting High Voltage to Low Voltage so Important?
1,000 volts is, and was, far too high a voltage for non-professionals to deal with, and 110 volts was chosen in the US and 230 volts in Europe. So why not just have the power stations just belt out either of those voltages?
Simple! Because any transmission line offers ohmic resistance.
In the simplified example below, let’s assume the power line presents R ohms, and compare the losses transmitting 100 volts at 100 amps versus the losses we see at 1,000 volts at 10 amps
Ohms law states that voltage equals current time resistance
Eq 1. V=I x R
We also know that power equals current times voltage
Eq 2. P = I x V
Substituting Eq 1. into Eq 2.
P = I x V = I x I x R
Eq 3. P = I2R
Equation 3 illustrates that the amount of power wasted in the power line is proportional to the square of the current
2- How Much Power Is Wasted In High Current Transmission?
So starting with equation 3, P = I2R, let’s see how much power is lost in the transmission line for 100 amps and 1,000 amps
At 100 amps, P = 1002 x R - That comes to 10,000 times R power wasted
At 10 amps, P = 102 x R - That comes to 100 times R power wasted
Dividing the top by the bottom yields 10,000 R / 100 R = 100
The results are clear. Transmitting high voltage at low current is far less wasteful than transmitting low voltage at high current
So, for over a century, much to Edison’s chagrin, the lion’s share of the world’s electrical power was transmitted as AC.
3- What is Pulsating DC Voltage?
If you were able to turn a DC voltage on and off in a periodic manner, you’d have pulsating DC, as illustrated below.
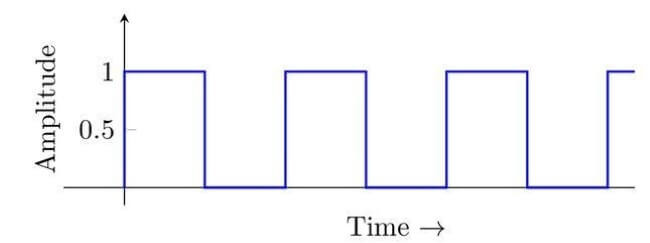
Pulsating DC. Image source (modified): Google
The reason why pulsating DC is so important to our story is that, in a manner similar to that for AC power, transformers can step up or step down pulsating DC power. Until very recently, it was impossible to do this without a tremendous loss of power.
4- Converting DC to Pulsating DC – What’s Changed?
In a word – Semiconductors! There are now semiconductor devices, such as insulated-gate bipolar transistors (IGBT) and Thyristors, that that can switch on and off many times per second with virtually no losses. More recently, purely silicon-based devices are being replaced by low-bandgap semiconductors built with Gallium Arsenide (GaAs) , Gallium Nitride (GaN ) and Silicon Carbide (SiC). These can handle even more power with even more efficiency.
So, thanks to this rolling revolution in semiconductor physics, we now have a way to efficiently convert high voltage power line DC to pulsating DC at the line’s terminus. The pulsating DC can be fed into a step-down transformer, which can efficiently convert it to any other low voltage AC format that is needed for home or industrial uses.
But, why would we want to? What are the advantages of high voltage DC power lines over high voltage AC power lines?
5- The Drawbacks of AC Power Lines
We now know that for either AC or DC, long-distance power lines must transmit power at low current and at very high voltages because of the power wasted by either modus in high current transmission. But, as illustrated below, at long distances even at 400,000 volts, AC power lines, still waste far more power than do DC power lines. - Why?
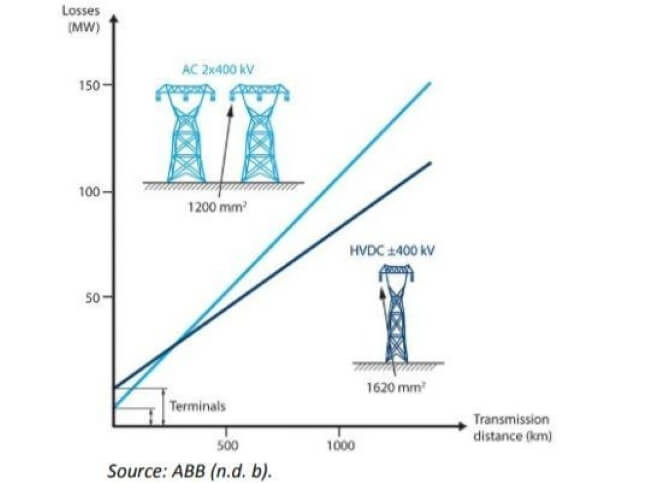
Image source: U.S. Energy Information Administration
6- The Skin Effect
This phenomenon occurs when AC voltage flows through a wire. It is absent in DC transmission.
For AC power conduction, the greatest current density occurs only on the surface of the wire conductor, while the core transmits very little of the power at all. Because most of the current pass through less of the wire’s area, electrically speaking, the cable is hollowed out, and less of the cable is doing any electrical work. And, because a “thinner’ cable offers more resistance than thicker a one, the same line will offer more resistance to AC power than to DC power.
7- Asynchronicity and the Smartgrid
As we advance toward smartgrid technology, different sources of power generation must be able to interconnect. The picture below illustrates two asynchronous AC power sources:
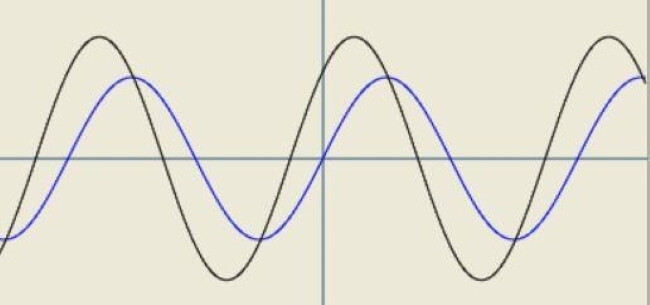
They are both cycling at the same frequency but are out of phase. They are also at different voltages. It would be impossible to directly connect these two, and the situation would be even more difficult if one were at a different frequency (50 cycles per second as opposed to 60 cycles per second.)
DC power is immune to this problem. So, even if the power lines are HVAC and not HVDC, conversion to HVDC will be absolutely necessary in order for disparate AC sources to work together on a smartgrid.
8- HVDC Power Lines Have Smaller Right-Of-Way Requirements
An HVDC power line requires only two physical cables, while a HVAC line requires six. As implied in the previous section’s illustration, this can mean two transmitting towers for HVAC as opposed to one for HVDC.
9- HVAC Unsuitable for Underwater Transmission
The nature of submarine cables is such that they present high parallel capacitance over distance. A “capacitor” only reacts to electrical signal during times of change, which is happening constantly with AC, and only during switch on and switch off for DC.
This will have the effect of sapping a large portion of AC power transmitted over underwater cables, but only effect HVDC during turn on and turn off.
10- HVDC Wastes Cable Carrying Capacity
In the representation below, the brown line is a DC signal, and the blue line is an AC signal.
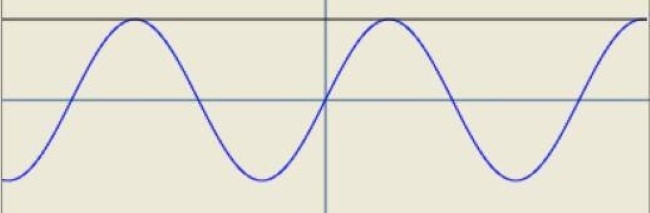
Even though the AC power peak is equal to the DC steady state, it is a well documented fact that the average power carried via the AC line is only 71% of that carried by the DC line.
However, the AC cable must be rated for the peak power, even though it carries only 71%. This is a tremendous waste inherent in all HVAC power lines.
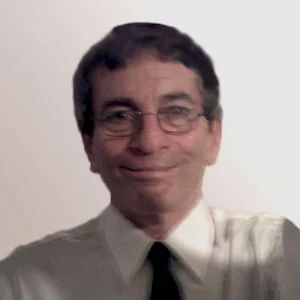
By Gary Elinoff
Gary Elinoff graduated from SUNY Stony Brook with a bachelor’s degree in physics and he also holds a master’s degree in electrical engineering from San Jose State University. Along the way, he was also awarded an MBA with a concentration in finance from Boston University. Now a professional science and engineering writer, he has worked in test engineering and as writer/editor for the electronic trade press.
Like this content, subscribe today
- New Products
- Top Sellers
- Gift Certificates
- Raspberry Pi
- Single Board Comp.
- Microcontrollers
- Machine Learning
- Prototyping Boards
- all development
- Environment
- all sensors
- 3D Printing
- Instruments
- Arts/Crafts Supplies
- LED & Illumination
- Buttons & Switches
- LCDs & OLEDs
- Cables & Wire
- all components
- Sewable Electronics
- E-Textile Power
- E-Textile Kits
- all e-textiles
- Motors & Drivers
- Robotics Kits
- all robotics
- GPS & GNSS
- Wireless Kits
- all wireless/IoT
- Audio Boards
- Audio Cables
- Audio Chips
Alternating Current (AC) vs. Direct Current (DC)
Thunderstruck!
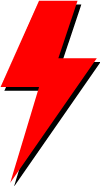
Where did the Australian rock band AC/DC get their name from? Why, Alternating Current and Direct Current, of course! Both AC and DC describe types of current flow in a circuit. In direct current (DC), the electric charge (current) only flows in one direction. Electric charge in alternating current (AC), on the other hand, changes direction periodically. The voltage in AC circuits also periodically reverses because the current changes direction.
Most of the digital electronics that you build will use DC. However, it is important to understand some AC concepts. Most homes are wired for AC, so if you plan to connect your Tardis music box project to an outlet, you will need to convert AC to DC. AC also has some useful properties, such as being able to convert voltage levels with a single component (a transformer), which is why AC was chosen as the primary means to transmit electricity over long distances.
What You Will Learn
- The history behind AC and DC
- Different ways to generate AC and DC
- Some examples of AC and DC applications
Recommended Reading
- What is Electricity
- What is a Circuit?
- Voltage, Current, Resistance, and Ohm's Law
- Electric Power
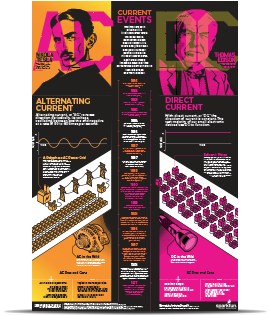
Alternating Current (AC)
Alternating current describes the flow of charge that changes direction periodically. As a result, the voltage level also reverses along with the current. AC is used to deliver power to houses, office buildings, etc.
Generating AC
AC can be produced using a device called an alternator. This device is a special type of electrical generator designed to produce alternating current.
A loop of wire is spun inside of a magnetic field, which induces a current along the wire. The rotation of the wire can come from any number of means: a wind turbine, a steam turbine, flowing water, and so on. Because the wire spins and enters a different magnetic polarity periodically, the voltage and current alternates on the wire. Here is a short animation showing this principle:
(Video credit: Khurram Tanvir)
Generating AC can be compared to our previous water analogy :
To generate AC in a set of water pipes, we connect a mechanical crank to a piston that moves water in the pipes back and forth (our "alternating" current). Notice that the pinched section of pipe still provides resistance to the flow of water regardless of the direction of flow.
AC can come in a number of forms, as long as the voltage and current are alternating. If we hook up an oscilloscope to a circuit with AC and plot its voltage over time, we might see a number of different waveforms. The most common type of AC is the sine wave. The AC in most homes and offices have an oscillating voltage that produces a sine wave.
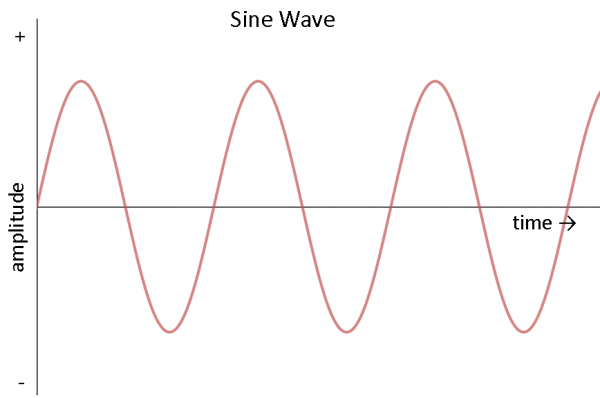
Other common forms of AC include the square wave and the triangle wave:
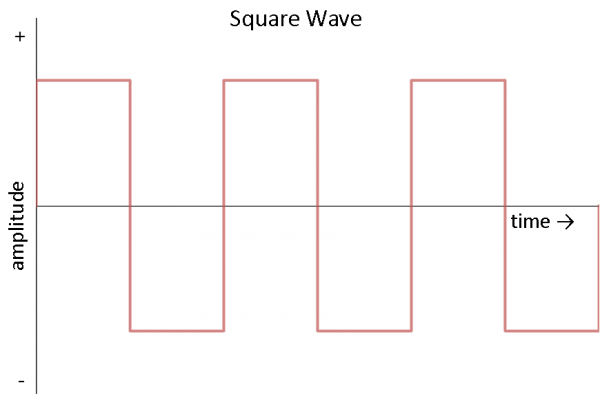
Square waves are often used in digital and switching electronics to test their operation.
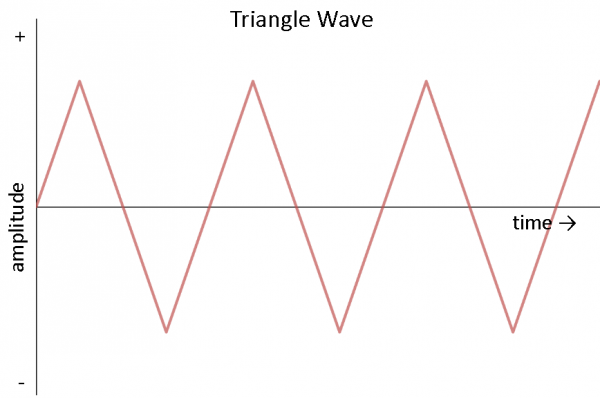
Triangle waves are found in sound synthesis and are useful for testing linear electronics like amplifiers.
Describing a Sine Wave
We often want to describe an AC waveform in mathematical terms. For this example, we will use the common sine wave. There are three parts to a sine wave: amplitude, frequency, and phase.
Looking at just voltage, we can describe a sine wave as the mathematical function:
V(t) is our voltage as a function of time, which means that our voltage changes as time changes. The equation to the right of the equals sign describes how the voltage changes over time.
V P is the amplitude . This describes the maximum voltage that our sine wave can reach in either direction, meaning that our voltage can be +V P volts, -V P volts, or somewhere in between.
The sin() function indicates that our voltage will be in the form of a periodic sine wave, which is a smooth oscillation around 0V.
2π is a constant that converts the freqency from cycles (in hertz) to angular frequnecy (radians per second).
f describes the frequency of the sine wave. This is given in the form of hertz or units per second . The frequency tells how many times a particular wave form (in this case, one cycle of our sine wave - a rise and a fall) occurs within one second.
t is our independent variable: time (measured in seconds). As time varies, our waveform varies.
φ describes the phase of the sine wave. Phase is a measure of how shifted the waveform is with respect to time. It is often given as a number between 0 and 360 and measured in degrees. Because of the periodic nature of the sine wave, if the wave form is shifted by 360° it becomes the same waveform again, as if it was shifted by 0°. For simplicity, we sill assume that phase is 0° for the rest of this tutorial.
We can turn to our trusty outlet for a good example of how an AC waveform works. In the United States, the power provided to our homes is AC with about 170V zero-to-peak (amplitude) and 60Hz (frequency). We can plug these numbers into our formula to get the equation (remember that we are assuming our phase is 0):
We can use our handy graphing calculator to graph this equation. If no graphing calculator is available we can use a free online graphing program like Desmos (Note that you might have to use 'y' instead of 'v' in the equation to see the graph).
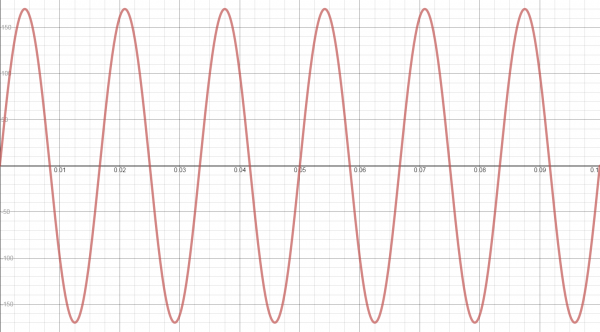
Notice that, as we predicted, the voltage rise up to 170V and down to -170V periodically. Additionally, 60 cycles of the sine wave occurs every second. If we were to measure the voltage in our outlets with an oscilloscope, this is what we would see ( WARNING: do not attempt to measure the voltage in an outlet with an oscilloscope! This will likely damage the equipment).
NOTE: You might have heard that AC voltage in the US is 120V. This is also correct. How? When talking about AC (since the voltage changes constantly), it is often easier to use an average or mean. To accomplish that, we use a method called "Root mean squared." (RMS). It is often helpful to use the RMS value for AC when you want to calculate electrical power . Even though, in our example, we had the voltage varying from -170V to 170V, the root mean square is 120V RMS.
Applications
Home and office outlets are almost always AC. This is because generating and transporting AC across long distances is relatively easy. At high voltages (over 110kV), less energy is lost in electrical power transmission. Higher voltages mean lower currents, and lower currents mean less heat generated in the power line due to resistance. AC can be converted to and from high voltages easily using transformers.
AC is also capable of powering electric motors. Motors and generators are the exact same device, but motors convert electrical energy into mechanical energy (if the shaft on a motor is spun, a voltage is generated at the terminals!). This is useful for many large appliances like dishwashers, refrigerators, and so on, which run on AC.
Direct Current (DC)
Direct current is a bit easier to understand than alternating current. Rather than oscillating back and forth, DC provides a constant voltage or current.
Generating DC
DC can be generated in a number of ways:
- An AC generator equipped with a device called a "commutator" can produce direct current
- Use of a device called a "rectifier" that converts AC to DC
- Batteries provide DC, which is generated from a chemical reaction inside of the battery
Using our water analogy again, DC is similar to a tank of water with a hose at the end.
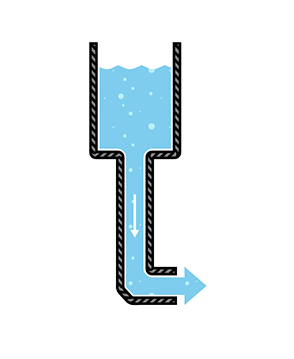
The tank can only push water one way: out the hose. Similar to our DC-producing battery, once the tank is empty, water no longer flows through the pipes.
Describing DC
DC is defined as the "unidirectional" flow of current; current only flows in one direction. Voltage and current can vary over time so long as the direction of flow does not change. To simplify things, we will assume that voltage is a constant. For example, we assume that a AA battery provides 1.5V, which can be described in mathematical terms as:
If we plot this over time, we see a constant voltage:
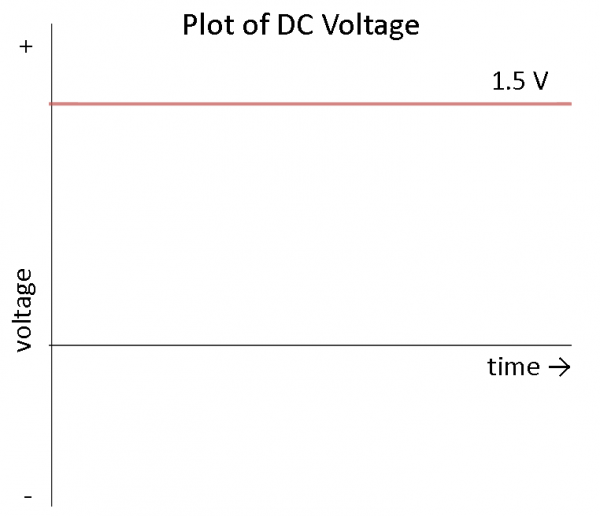
What does this mean? It means that we can count on most DC sources to provide a constant voltage over time. In reality, a battery will slowly lose its charge, meaning that the voltage will drop as the battery is used. For most purposes, we can assume that the voltage is constant.
Almost all electronics projects and parts for sale on SparkFun run on DC. Everything that runs off of a battery, plugs in to the wall with an AC adapter , or uses a USB cable for power relies on DC. Examples of DC electronics include:
- Cell phones
- The LilyPad-based D&D Dice Gauntlet
- Flat-screen TVs (AC goes into the TV, which is converted to DC)
- Flashlights
- Hybrid and electric vehicles
Battle of the Currents
Almost every home and business is wired for AC. However, this was not an overnight decision. In the late 1880s, a variety of inventions across the United States and Europe led to a full-scale battle between alternating current and direct current distribution.
In 1886, Ganz Works, an electric company located in Budapest, electrified all of Rome with AC. Thomas Edison, on the other hand, had constructed 121 DC power stations in the United States by 1887. A turning point in the battle came when George Westinghouse, a famous industrialist from Pittsburgh, purchased Nikola Tesla's patents for AC motors and transmission the next year.
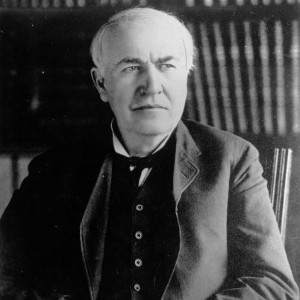
Thomas Edison (Image courtesy of biography.com )
In the late 1800s, DC could not be easily converted to high voltages. As a result, Edison proposed a system of small, local power plants that would power individual neighborhoods or city sections. Power was distributed using three wires from the power plant: +110 volts, 0 volts, and -110 volts. Lights and motors could be connected between either the +110V or 110V socket and 0V (neutral). 110V allowed for some voltage drop between the plant and the load (home, office, etc.).
Even though the voltage drop across the power lines was accounted for, power plants needed to be located within 1 mile of the end user. This limitation made power distribution in rural areas extremely difficult, if not impossible.
With Tesla's patents, Westinghouse worked to perfect the AC distribution system. Transformers provided an inexpensive method to step up the voltage of AC to several thousand volts and back down to usable levels. At higher voltages, the same power could be transmitted at much lower current, which meant less power lost due to resistance in the wires. As a result, large power plants could be located many miles away and service a greater number of people and buildings.
Edison's Smear Campaign
Over the next few years, Edison ran a campaign to highly discourage the use of AC in the United States, which included lobbying state legislatures and spreading disinformation about AC. Edison also directed several technicians to publicly electrocute animals with AC in an attempt to show that AC was more dangerous than DC. In attempt to display these dangers, Harold P. Brown and Arthur Kennelly, employees of Edison, designed the first electric chair for the state of New York using AC.
The Rise of AC
In 1891, the International Electro-Technical Exhibition was held in Frankfurt, Germany and displayed the first long distance transmission of three-phase AC, which powered lights and motors at the exhibition. Several representatives from what would become General Electric were present and were subsequently impressed by the display. The following year, General Electric formed and began to invest in AC technology.
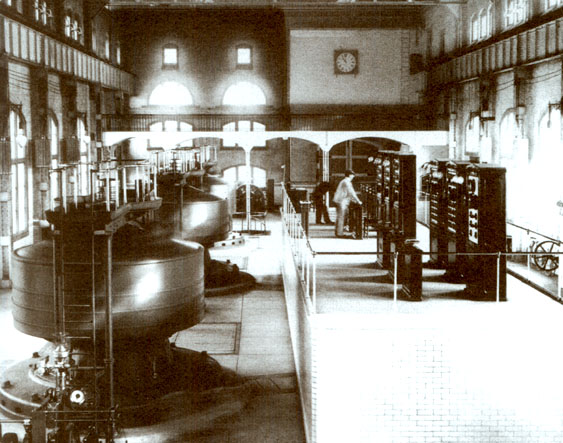
Edward Dean Adams Power Plant at Niagara Falls, 1896 (Image courtesy of teslasociety.com )
Westinghouse won a contract in 1893 to build a hydroelectric dam to harness the power of Niagara falls and transmit AC to Buffalo, NY. The project was completed on November 16, 1896 and AC power began to power industries in Buffalo. This milestone marked the decline of DC in the United States. While Europe would adopt an AC standard of 220-240 volts at 50 Hz, the standard in North America would become 120 volts at 60 Hz.
High-Voltage Direct Current (HVDC)
Swiss engineer René Thury used a series of motor-generators to create a high-voltage DC system in the 1880s, which could be used to transmit DC power over long distances. However, due to the high cost and maintenance of the Thury systems, HVDC was never adopted for almost a century.
With the invention of semiconductor electronics in the 1970s, economically transforming between AC and DC became possible. Specialized equipment could be used to generate high voltage DC power (some reaching 800 kV). Parts of Europe have begun to employ HVDC lines to electrically connect various countries.
HVDC lines experience less loss than equivalent AC lines over extremely long distances. Additionally, HVDC allows different AC systems (e.g. 50 Hz and 60 Hz) to be connected. Despite its advantages, HVDC systems are more costly and less reliable than the common AC systems.
In the end, Edison, Tesla, and Westinghouse may have their wishes come true. AC and DC can coexist and each serve a purpose.
Resources and Going Further
You should now have a good understanding of the differences between AC and DC. AC is easier to transform between voltage levels, which makes high-voltage transmission more feasible. DC, on the other hand, is found in almost all electronics. You should know that the two do not mix very well, and you will need to transform AC to DC if you wish to plug in most electronics into a wall outlet. With this understanding, you should be ready to tackle some more complex circuitry and concepts, even if they contain AC.
Take a look at the following tutorials when you are ready to dive deeper into the world of electronics:
- Series and Parallel Circuits
- Logic Levels
- How to Use a Multimeter
- How to Power a Project
Interested in learning more foundational topics?
See our Engineering Essentials page for a full list of cornerstone topics surrounding electrical engineering.
Take me there!
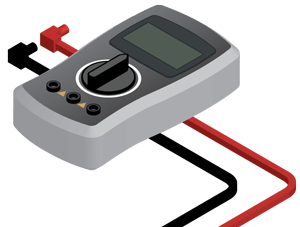
- Electrical Engineering
- Creative Commons tutorials are CC BY-SA 4.0
- Your Account
- Undergraduate
- Postdoctoral Programs
- Future Engineers
- Professional Education
- Open Access
- Global Experiences
- Student Activities
- Leadership Development
- Graduate Student Fellowships
- Aeronautics and Astronautics
- Biological Engineering
- Chemical Engineering
- Civil and Environmental Engineering
- Electrical Engineering and Computer Science
- Institute for Medical Engineering and Science
- Materials Science and Engineering
- Mechanical Engineering
- Nuclear Science and Engineering
- Industry Collaborations
- Engineering in Action
- In The News
- Video Features
- Newsletter: The Infinite
- Ask an Engineer
- Facts and Figures
- Diversity, Equity & Inclusion
- Staff Spotlights
- Commencement 2023

Ask An Engineer
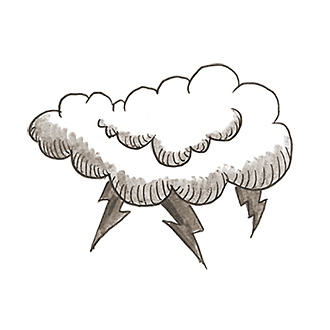
Related Questions
- Why can’t fusion energy solve the global energy crisis?
- Is it possible to collect energy from foot traffic?
- What is a short circuit?
- How many wind turbines would it take to power all of New York City?
- What happens to electricity when nothing is plugged into an outlet?
- What is “clean” coal?
- Why do the products of a nuclear fission reaction in uranium have three neutrons but not three protons?
- How does a battery work?
- Which is more likely to happen first: solar panels on every home, or giant solar power plants?
- Can we calculate the efficiency of a natural photosynthesis process?
What’s the difference between AC and DC?
One looks like a straight line, the other a wave; together, they power your laptop…
Alternating current (AC) and direct current (DC) are notable for inspiring the name of an iconic metal band, but they also happen to sit right at the center of the modern world as we know it. AC and DC are different types of voltage or current used for the conduction and transmission of electrical energy. Quick — think of five things you do or touch in a day that do not involve electricity in any way, were not produced using electricity, and are not related to your own body’s internal uses of electricity… Nice try, but no way, you can’t do it. (Or send us a list if you think you can; we’ll check it.)
Electrical current is the flow of charged particles, or specifically in the case of AC and DC, the flow of electrons. According to Karl K. Berggren, professor of electrical engineering at MIT, the fundamental difference between AC and DC is the direction of flow. DC is constant and moves in one direction. “A simple way to visualize the difference is that, when graphed, a DC current looks like a flat line, whereas the flow of AC on a graph makes a sinusoid or wave-like pattern,” says Berggren. “This is because AC changes over time in an oscillating repetition — the up curve indicates the current flowing in a positive direction and the down curve signifies the alternate cycle where the current moves in a negative direction. This back and forth is what gives AC its name.”
Leaving aside lines and graphs for a moment, Berggren offers another way to distinguish between AC and DC by looking at how they work in the devices we use. The lamp next to your bed, for example, uses AC. This is because the source of the current came from far away, and the wave-like motion of the current makes it an efficient traveler. If you happen to be a read-by-flashlight kind of person, you are a consumer of DC power. A typical battery has negative and positive terminals, and the electrical charge (it’s those electrons) moves in one direction from one to the other at a steady rate (the straight line on the graph).
Interestingly, if you’re reading this on a laptop, you are actually using both kinds of current. The nozzle-shaped plug that goes into your computer delivers a direct current to the computer’s battery, but it receives that charge from an AC plug that goes into the wall. The awkward little block that’s in between the wall plug and your computer is a power adapter that transforms AC to DC.
Berggren explains that AC became popular in the late 19th century because of its ability to efficiently distribute power at low voltages. Initially, power is conducted at very high voltages. In order to get these high voltages down to the low voltages necessary to power, say, a household light bulb, it’s necessary to transform the current. A transformer, which is basically two loops of wire, gets AC down from hundreds of thousands of volts to distributions of reasonable voltages (in the hundreds) to power most day to day electronics. The ability to transform voltages from AC meant that it was possible to transmit power much more efficiently across the country.
According to Berggren, there’s a funny history of rivalry between AC and DC. In the later 19th century, there was a giant war between Edison and Westinghouse over AC and DC. Edison had patents in place that made him invested in the widespread use of DC. He set out to convince the world that DC was superior for the transmission and distribution of power. He resorted to crazy demonstrations like killing large animals with AC in an attempt to prove its terrible dangers. For a time, he was successful and most municipalities utilized local power plants with DC supply. However, getting power to less populated, rural communities all over the country with DC proved very inefficient, so Westinghouse ultimately won out and AC became the dominant power source.
Thanks to 10-year-old Graham from Providence, RI, for this question.
Posted: September 17, 2013
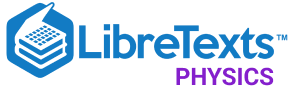
- school Campus Bookshelves
- menu_book Bookshelves
- perm_media Learning Objects
- login Login
- how_to_reg Request Instructor Account
- hub Instructor Commons
- Download Page (PDF)
- Download Full Book (PDF)
- Periodic Table
- Physics Constants
- Scientific Calculator
- Reference & Cite
- Tools expand_more
- Readability
selected template will load here
This action is not available.
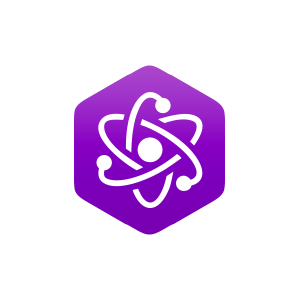
20.5: Alternating Current versus Direct Current
- Last updated
- Save as PDF
- Page ID 2683
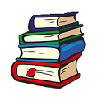
Learning Objectives
By the end of this section, you will be able to:
- Explain the differences and similarities between AC and DC current.
- Calculate rms voltage, current, and average power.
- Explain why AC current is used for power transmission.
Alternating Current
Most of the examples dealt with so far, and particularly those utilizing batteries, have constant voltage sources. Once the current is established, it is thus also a constant. Direct current (DC) is the flow of electric charge in only one direction. It is the steady state of a constant-voltage circuit. Most well-known applications, however, use a time-varying voltage source. Alternating current (AC) is the flow of electric charge that periodically reverses direction. If the source varies periodically, particularly sinusoidally, the circuit is known as an alternating current circuit. Examples include the commercial and residential power that serves so many of our needs. Figure \(\PageIndex{1}\) shows graphs of voltage and current versus time for typical DC and AC power. The AC voltages and frequencies commonly used in homes and businesses vary around the world.
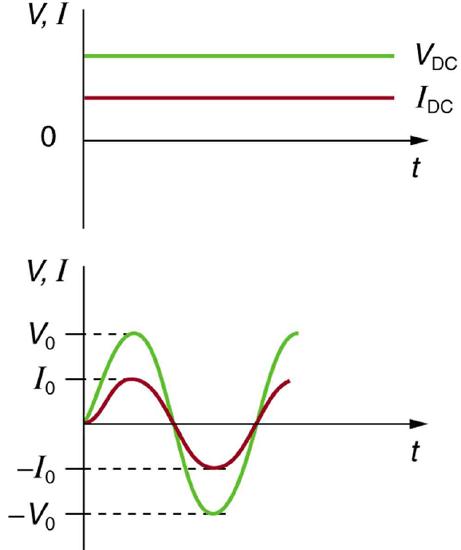
Figure \(\PageIndex{2}\) shows a schematic of a simple circuit with an AC voltage source. The voltage between the terminals fluctuates as shown, with the AC voltage given by \[V = V_{0} sin 2 \pi ft, \label{20.6.1}\] where \(V\) is the voltage at time \(t\), \(V_{0}\), \(V_{0}\) is the peak voltage, and \(f\) is the frequency in hertz. For this simple resistance circuit, \(I = V/R\), and so the AC current is
\[I = I_{0} sin 2 \pi ft, \label{20.6.2}\]
where \(I\) is the current at time \(t\), and \(I_{0} = V_{0} / R\) is the peak current. For this example, the voltage and current are said to be in phase, as seen in Figure \(\PageIndex{1b}\).
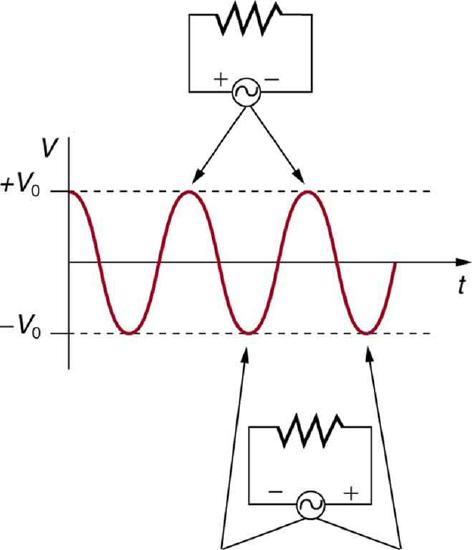
Current in the resistor alternates back and forth just like the driving voltage, since \(I = V / R\). If the resistor is a fluorescent light bulb, for example, it brightens and dims 120 times per second as the current repeatedly goes through zero. A 120-Hz flicker is too rapid for your eyes to detect, but if you wave your hand back and forth between your face and a fluorescent light, you will see a stroboscopic effect evidencing AC. The fact that the light output fluctuates means that the power is fluctuating. The power supplied is \(P = IV\). Using the expressions for \(I\) and \(V\) above, we see that the time dependence of power is \(P = I_{0}V_{0}sin^{2}2\pi ft\), as shown in Figure \(\PageIndex{3}\).
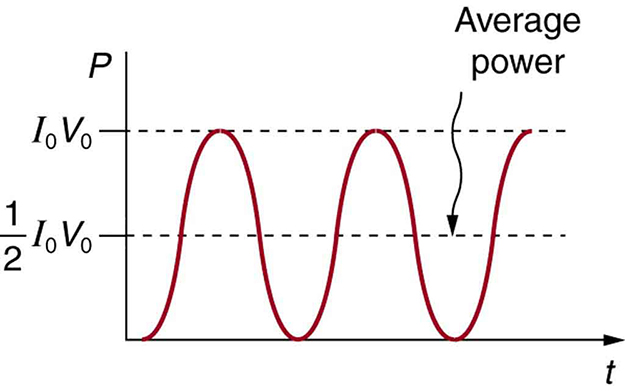
Making Connections: Take-Home Experiment—AC/DC Lights
Wave your hand back and forth between your face and a fluorescent light bulb. Do you observe the same thing with the headlights on your car? Explain what you observe. Warning: Do not look directly at very bright light.
We are most often concerned with average power rather than its fluctuations—that 60-W light bulb in your desk lamp has an average power consumption of 60 W, for example. As illustrated in Figure 3, the average power \(P_{ave}\) is \[P_{ave} = \frac{1}{2}I_{0}V_{0}. \label{20.6.3}\] This is evident from the graph, since the areas above and below the \(\left(1/2\right)I_{0}V_{0}\) line are equal, but it can also be proven using trigonometric identities. Similarly, we define an average or rms current \(I_{rms}\) and average or rms voltage \(V_{rms}\) to be, respectively,
\[I_{rms} = \frac{I_{0}}{\sqrt{2}}\label{20.6.4}\]
\[V_{rms} = \frac{V_{0}}{\sqrt{2}}.\label{20.6.5}\]
where rms stands for root mean square, a particular kind of average. In general, to obtain a root mean square, the particular quantity is squared, its mean (or average) is found, and the square root is taken. This is useful for AC, since the average value is zero. Now, \[P_{ave} = I_{rms}V_{rms}, \label{20.6.6}\] which gives
\[P_{ave} = \frac{I_{0}}{\sqrt{2}} \cdot \frac{V_{0}}{\sqrt{2}} = \frac{1}{2}I_{0}V_{0}, \label{20.6.7}\]
as stated above. It is standard practice to quote \(I_{rms}\), \(V_{rms}\), and \(P_{ave}\) rather than the peak values. For example, most household electricity is 120 V AC, which means that \(V_{rms}\) is 120 V. The common 10-A circuit breaker will interrupt a sustained \(I_{rms}\) greater than 10 A. Your 1.0-kW microwave oven consumes \(P_{ave} = 1.0 kW\), and so on. You can think of these rms and average values as the equivalent DC values for a simple resistive circuit.
To summarize, when dealing with AC, Ohm’s law and the equations for power are completely analogous to those for DC, but rms and average values are used for AC. Thus, for AC, Ohm’s law is written
\[I_{rms} = \frac{V_{rms}}{R}.\label{20.6.8}\]
The various expressions for AC power \(P_{ave}\) are
\[P_{ave} = I_{rms}V_{rms},\label{20.6.9}\]
\[P_{ave} = \frac{V^{2}_{rms}}{R},\label{20.6.10}\]
\[P_{ave} = I^{2}_{rms}R.\label{20.6.11}\]
Example \(\PageIndex{1}\): Peak Voltage and Power for AC
(a) What is the value of the peak voltage for 120-V AC power?
We are told that \(V_{rms}\) is 120V and \(P_{ave}\) is 60.0 W. We can use \(V_{rms} = \frac{V_{0}}{\sqrt{2}}\) to find the peak voltage, and we can manipulate the definition of power to find the peak power from the given average power.
Solution Solving the equation \(V_{rms}=\frac{V_{0}}{\sqrt{2}}\) for the peak voltage \(V_{0}\) and substituting the known value for \(V_{rms}\) gives \[V_{0} = \sqrt{2} V_{rms} = 1.414\left(120 V\right) = 170 V.\]
This means that the AC voltage swings from 170 V to \(-170 V\) and back 60 times every second. An equivalent DC voltage is a constant 120 V.
(b) What is the peak power consumption rate of a 60.0-W AC light bulb?
Peak power is peak current times peak voltage. Thus, \[P_{0} = I_{0}V_{0} = 2\left(\frac{1}{2} I_{0} V_{0} \right) = 2P_{ave}.\] We know the average power is 60.0 W, and so \[P_{0} = 2\left(60.0 W\right) = 120 W.\]
So the power swings from zero to 120 W one hundred twenty times per second (twice each cycle), and the power averages 60 W.
Why Use AC for Power Distribution?
Most large power-distribution systems are AC. Moreover, the power is transmitted at much higher voltages than the 120-V AC (240 V in most parts of the world) we use in homes and on the job. Economies of scale make it cheaper to build a few very large electric power-generation plants than to build numerous small ones. This necessitates sending power long distances, and it is obviously important that energy losses en route be minimized. High voltages can be transmitted with much smaller power losses than low voltages, as we shall see. (See Figure 4.) For safety reasons, the voltage at the user is reduced to familiar values. The crucial factor is that it is much easier to increase and decrease AC voltages than DC, so AC is used in most large power distribution systems.
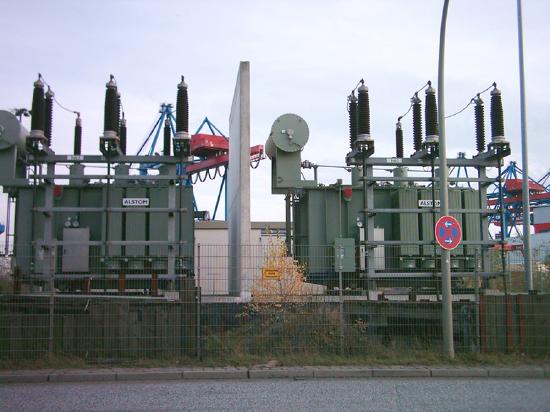
Example \(\PageIndex{2}\): Power losses are less for high-voltage transmission
(a) What current is needed to transmit 100 MW of power at 200 kV?
We are given \(P_{ave} = 100 MW\), \(V_{rms} = 200 kV\), and the resistance of the lines is \(R = 1.00 \Omega\). Using these givens, we can find the current flowing (from \(P = IV\) ) and then the power dissipated in the lines (\(P = I^{2}R\)), and we take the ratio to the total power transmitted.
To find the current, we rearrange the relationship \(P_{ave} = I_{rms}V_{rms}\) and substitute known values. This gives \[I_{rms} = \frac{P_{ave}}{V_{rms}} = \frac{100 \times 10^{6} W}{200 \times 0^{3} V} = 500A .\]
(b) What is the power dissipated by the transmission lines if they have a resistance of \(1.00 \Omega\)?
Knowing the current and given the resistance of the lines, the power dissipated in them is found from \(P_{ave} = I^{2}_{rms}R.\). Substituting the known value gives \[P_{ave} = I^{2}_{rms}R = \left(500 A\right)^{2} \left(1.00 \Omega\right) = 250 kW.\]
(c) What percentage of the power is lost in the transmission lines?
The percent loss is the ratio of this lost power to the total or input power, multiplied by 100: \[% loss = \frac{250kW}{100 MW} \times 100 = 0.250 %.\]
One-fourth of a percent is an acceptable loss. Note that if 100 MW of power had been transmitted at 25 kV, then a current of 4000 A would have been needed. This would result in a power loss in the lines of 16.0 MW, or 16.0% rather than 0.250%. The lower the voltage, the more current is needed, and the greater the power loss in the fixed-resistance transmission lines. Of course, lower-resistance lines can be built, but this requires larger and more expensive wires. If superconducting lines could be economically produced, there would be no loss in the transmission lines at all. But, as we shall see in a later chapter, there is a limit to current in superconductors, too. In short, high voltages are more economical for transmitting power, and AC voltage is much easier to raise and lower, so that AC is used in most large-scale power distribution systems.
It is widely recognized that high voltages pose greater hazards than low voltages. But, in fact, some high voltages, such as those associated with common static electricity, can be harmless. So it is not voltage alone that determines a hazard. It is not so widely recognized that AC shocks are often more harmful than similar DC shocks. Thomas Edison thought that AC shocks were more harmful and set up a DC power-distribution system in New York City in the late 1800s. There were bitter fights, in particular between Edison and George Westinghouse and Nikola Tesla, who were advocating the use of AC in early power-distribution systems. AC has prevailed largely due to transformers and lower power losses with high-voltage transmission.
PHET EXPLORATIONS: GENERATOR
Generate electricity with a bar magnet! Discover the physics behind the phenomena by exploring magnets and how you can use them to make a bulb light.
Figure \(\PageIndex{5}\): Generator
- Direct current (DC) is the flow of electric current in only one direction. It refers to systems where the source voltage is constant.
- The voltage source of an alternating current (AC) system puts out \(V= V_{0} \sin{2\pi} ft\), where \(V\) is the voltage at time \(t\), \(V_{0}\) is the peak voltage, and \(f\) is the frequency in hertz.
- In a simple circuit, \(I = V/R\) and AC current is \(I = I_{0} \sin{2\pi}ft\), where \(I\) is the current at time \(t\), and \(I_{0} = V_{0}/R\) is the peak current.
- The average AC power is \(P_{ave} = \frac{1}{2} I_{0}V_{0}\).
- Average (rms) current \(I_{rms}\) and average (rms) voltage \(V_{rms}\) and \(I_{rms} = \frac{I_{0}}{\sqrt{2}}\) and \(V_{rms} = \frac{V_{0}}{\sqrt{2}}\), where rms stands for root mean square.
- Thus, \(P_{ave} = I_{rms}V_{rms}\).
- Ohm's law for AC is \(I_{rms} = \frac{V_{rms}}{R}\).
- Expressions for the average power of an AC circuit are \(P_{ave} = I_{rms}V_{rms}\), \(P_{ave} = \frac{V_{rms}^{2}}{R}\), and \(P_{ave} = I_{rms}^{2}R\), analogous to the expressions for DC circuits.
Claimed by Keenan Witsken
- 1.1 How It Works
- 2.1 Direct Current (DC)
- 2.2 Alternating Current (AC)
- 3 Connectedness
- 5 References
The Main Idea
Direct Current, or DC, is the name given to a steady constant current in a circuit that consists of a battery and a type of resistor. DC has a steady flow of electrons through a circuit and is used to power items such as a laptop, iPod, flashlight, radio, and almost any other electronic device. Basically, items that have batteries in them use DC power. Alternating Current, or AC, uses sinusoidally alternating current that flickers at extremely high speeds. This current moves back and forth throughout the object being powered, such as a lamp or a refrigerator.
How It Works
Basically, a Direct Current (DC) power source consists of a battery and a resistor. This circuit is unidirectional, which means that the current can only travel on one direction. An Alternating Current (AC) is a bit more complicated. Rather than only going in one direction, AC current travels back and forth in a system in a sinusoidal pattern, meaning that between every wavelength, there is a time when the current is stopped. AC usually travels at about 60 Hz which means that objects like the lamps in our office are actually flickering on and off at such a fast pace that we don't even notice the periods when the lamp flickers off. Usually the way that such an AC circuit works is that there is a magnet that spins close to the circuit so that when the positive end of the magnet is facing upwards and the negative end is facing downwards, the electrons are flowing upwards throughout the circuit. Likewise, when the positive end of the magnet is facing downwards and the negative end is upwards, the electrons flow downwards. When this magnet spins and alternates whether the positive end is facing up or down, the electrons switch off going up or down in the circuit. This creates the sinusoidal wave that I mentioned above. The magnet spins so quickly (about 3600 rpm) that it is extremely difficult to tell that the light is flickering. Sometimes, if you wave your hand in front of a fluorescent light bulb you can see the flickering.
Advantages and Disadvantages
Direct current (dc).
Direct Current power is more efficient when transferring power to smaller loads, such as a laptop or a cellphone. A battery is more effective and also doesn't have skips in the power supply that an AC has since its voltage is sinusoidal. Also, using AC requires a transformer when transferring power of large distances. Also a DC does not introduce reactance in the line which transfers to higher power transfer in the line. This leads to lower cost.
Alternating Current (AC)
Alternating Current power is used in almost all home and office outlets. This is because it is more efficient when transferring power over large distances. Since P=IV, alternating current allows the supplier to greatly increase the voltage and lower the current so that there is no loss in energy through heat. If the current was very high, there would be a large loss of energy through overheating. Also, using DC only allows for buildings to be powered within a one mile radius which is ineffective when trying to power a whole town.
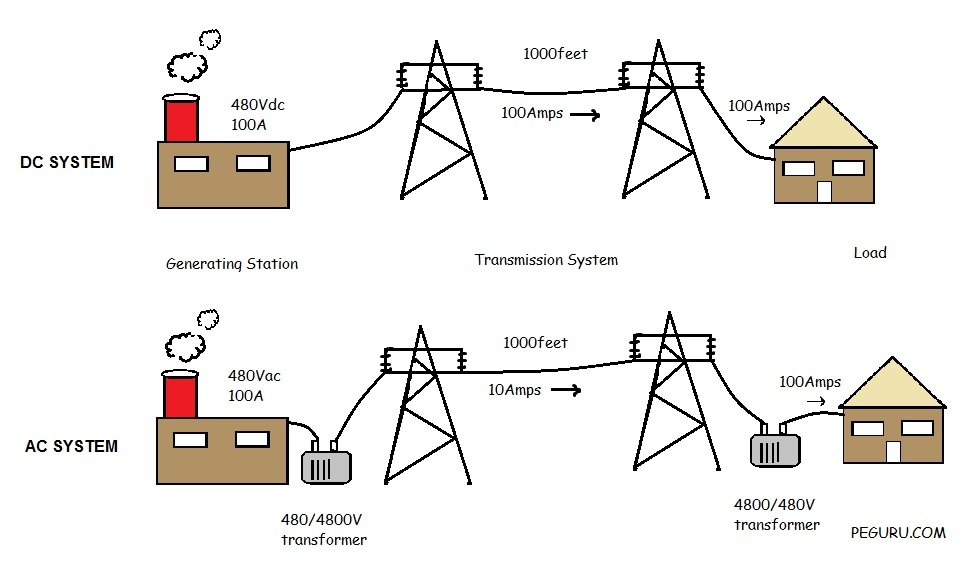
Connectedness
- How is this topic connected to something that you are interested in?
I find this topic very interesting because I have studied batteries in the past but also have a large fascination with a lot of the work that Thomas Edison invented. Thomas Edison was one of the innovative founders of General Electric and my father worked for General Electric for over 20 years. I would like to work for GE because of the innovative ideas and the learning programs that it includes in its co-op program.
- How is it connected to your major?
All of this is extrememly connected to my major because on the DC/Thomas Edison side of the spectrum, I aspire to work for General Electric. Being a mechanical engineer, General Electric offers one of the most prestigious mechanical engineering co-ops and provides one of the best learning programs for people studying mechanical engineering. Secondly, on the AC/Nikola Tesla side of things, I am fascinated with cars. I have had the opportunity to study batteries in the past and am amazed by the way in which the progression of technology is very closely related to the advancement of batteries. Being a mechanical engineer, studying the Tesla car and its motor is intriguing. Rather than using gasoline, the Tesla motor is run by an AC energy source. As explained above, this is because it is more practical since a DC energy source causes too much lost power through heat transfer.
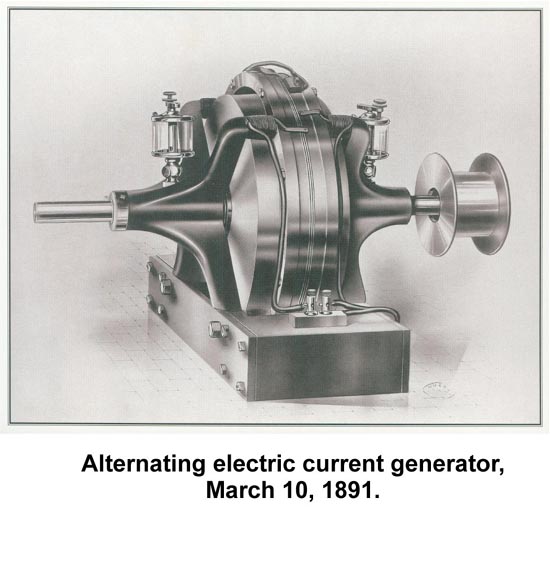
- Is there an interesting industrial application?
People do not understand how important this topic plays in their everyday lives. Houses and office building all over the world use AC power sources through outlets to charge their mobile devices, laptops, and more. Batteries play a huge role in the advancement of technology and most devices that use batteries likely use the DC energy source. Other objects such as lamps and refrigerators use and Alternating Current.
Thomas Edison was the first to come up with DC in the way in which he tried to supply power to towns all over the US and Europe using DC energy sources. Nikola Tesla also wanted to supply energy to the world but through the use of AC rather than DC. Thomas Edison took Nikola Tesla under his wing in the late 1800s and both of these great minds brainstormed great ways to power America. When Thomas Edison started his plan to power towns with DC, Nikola Tesla argued that AC current was a better path to take. After an argument took place, Nikola was fired and Edison continued his plan to provide energy using DC power. Although AC is used to power houses all over the world today, during the late 1800s, it was a huge battle between AC and DC power to decide which energy source should take over. Thomas Edison even created advertisements and spread rumors about how AC currents were far more dangerous and showed this through electrocuting animals using AC. Once it was found that it is much easier to transfer AC long distances, AC became the common household energy source. This is because DC can only be used to power homes and buildings within one mile of the energy plant.
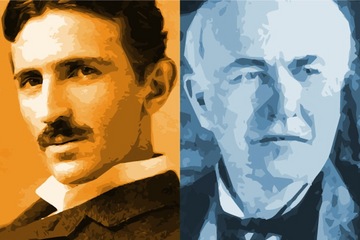
AC vs DC Image: http://blog.gogreensolar.com/2015/02/ac-vs-dc-breakers.html
Thomas Edison-Nikola Tesla Image: http://www.livescience.com/46739-tesla-vs-edison-comparison.html
Tesla Motor Image: http://www.teslasociety.com/hall_of_fame.htm
Industrial Usage Image: http://peguru.com/2011/03/ac-dc-power/
AC vs DC GIF: http://www.physicsbook.gatech.edu/File:Ac-vs-dc-o.gif
- Which Category did you place this in?
Navigation menu
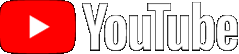
Navigation menu
There are two types of electrical current, AC and DC . One flows back and forth alternating directions: alternating current . The other flows consistently in the same direction direct current . This page discusses the differences between the two, along with the advantages each carries along with it.
Brief summary of AC and DC
- Alternating current is produced within most types of power plants by spinning generators . The direction of current reverses, or alternates, 50-60 times per second depending on a country's standards. [1] Alternating current is the current that travels through power lines and comes through the power outlets found throughout a home or building. There are various reasons why AC was the current of choice to perform this task, which will be discussed below.
- Direct current is produced by power sources like batteries , fuel cells , and solar panels . Such power sources have two terminals that are positive and negative respectively, which creates a relatively constant voltage for electrons to flow through. Current always flows in the same direction between these two terminals. [1]
Figure 1. An animation from a PhET simulation of alternating current which has been slowed down considerably. [2]
Figure 2. An animation from a PhET simulation of direct current which has been slowed down considerably. [3]
Advantages of each
Alternating current uses of varying voltage and flow of electrons within a conductor . Direct current flows in one direction and with a relatively constant current (charge flowing by a point per unit time). The way each can be manipulated, however, is what is important, and provides clear advantages for certain applications among the two.
Advantages of AC
AC is the current of choice for power plants and the electrical grid as a whole. When a plug is connected to an electrical outlet , alternating current comes out, providing power to countless devices like light bulbs and refrigerators . AC is preferred for this application because:
Resistance reduces the energy transmitted in a wire. By increasing the voltage on the wires to very high voltages for long distance transmission, this loss can be reduced. The loss of power ( [math]P_{lost}[/math] ) is given by the equation: [4]
- [math]I[/math] is the current in amperes
- [math]R[/math] is the resistance in ohms
Increasing the voltages the grid transmits electricity reduces this lost power. As the voltage gets higher, the current decreases proportionally because the transmitted electrical power (energy per unit time) remains the same. For example, if the voltage is increased by a factor of 100, the current must decrease by a factor of 100 and the resulting power lost will be decreased by 100 2 = 10000. However there is a limit, being that at extremely high voltages (2000 kV) the electricity begins to discharge resulting in high losses. [4]
Efficient transmission saves power companies and consumers a lot of money, which helps reduce pollution since power plants do not need to make up for lost electricity by using more fuel .
Other advantages of AC include: [5]
- Low maintenance costs of high speed AC motors.
- Easy to interrupt the current (ie. with a circuit breaker ) due to the current going to zero naturally every 1/2 cycle. For example, a circuit breaker can interrupt about 1/20th as much DC as AC current.
Advantages of DC
A big advantage of direct current is that it is easier to change the speed of a DC electric motor than it is for an AC one. This is useful in many applications, such as electric and hybrid cars . [5]
Direct current is used in essentially all consumer electronics, since transistors (the building blocks of modern electronics) rely on it to operate. Devices that use DC current include cell phones , laptops, TVs and much more.
Direct current may also be used to transmit electricity with even greater efficiency than alternating current over extremely large distances by use of HVDC transmission (high-voltage direct current). Along with higher efficiency, HVDC also allows for different AC systems (ie. 50 Hz and 60 Hz) to be connected. [6]
For Further Reading
For further information please see the related pages below:
- Electrical transmission
- Electrical grid
- Electrical generation
- Distribution grid
- Or explore a random page!
- ↑ 1.0 1.1 How Stuff Works. (Accessed December 30, 2015). Direct Current Versus Alternating Current [Online], Available: http://science.howstuffworks.com/electricity8.htm
- ↑ http://phet.colorado.edu/sims/circuit-construction-kit/circuit-construction-kit-ac_en.jnlp
- ↑ 4.0 4.1 R. Paynter and B.J. Boydell, "Transmission Lines and Substations" in Introduction to Electricity , 1st ed., Upper Saddle River, NJ: Pearson, 2011, ch.25, sec.3, pp.1102-1104
- ↑ 5.0 5.1 Private communication with M. Pigman power engineer for Tacoma Power, Sept. 17th, 2015.
- ↑ Spark Fun. (Accessed December 30, 2015). Alternating Current vs. Direct Current [Online], Available: https://learn.sparkfun.com/tutorials/alternating-current-ac-vs-direct-current-dc
Intelligent insights & conversations with global power industry professionals

The Energy Collective Group
This group brings together the best thinkers on energy and climate. Join us for smart, insightful posts and conversations about where the energy industry is and where it is going.
- AC vs. DC Powerlines and the Electrical Grid

- Member since 2010
- 71 items added with 44,840 views
- Comment (8)
- Apr 2, 2013 Jul 7, 2018 12:49 am GMT
- 41855 views

There are different trade-offs for AC versus DC power transmission. Voltage can readily be taken up to about 765,000 volts (765 kV) for an AC powerline (this is the current maximum AC voltage in the US) but beyond that, power dissipation through dielectric loss becomes significant. (Dielectric losses are caused when dipoles in matter align with a changing local electric field. As the polar structures turn to follow the field, the movement causes local heating. This is the basis of microwave ovens. The dielectric loss during transmission is equal to the total heat that is generated in materials around the powerlines due to induced motions of electric dipoles.) At high voltage, non-resistive power dissipation via dielectric losses (for AC only) and/or through corona discharge (for both AC and DC) becomes severe. Voltage for DC overhead powerlines can be taken up to higher voltage than the maximum practical AC voltage; at present the worldwide maximum is ± 800 kV for HVDC lines. Note that the way that voltage is reported for AC vs. DC powerlines is different; a ± 800 kV DC powerline has 1600 kV conductor to conductor (800 kV conductor to ground), whereas AC voltage refers to the conductor to conductor root mean square, or “rms” voltage; roughly speaking AC rms voltage is comparable to the line-to-line voltage in DC in terms of transmission capacity. In effect, HVDC voltage can go about twice as high as HVAC voltage, which explains most of the advantage of overhead HVDC lines compared to overhead HVAC lines.
Wire diameter is limited for AC transmission lines due to the “ skin effect ” that prevents an AC current from penetrating to the center of a large wire, whereas a DC line can be arbitrarily thick. At 60 Hz, the skin effect becomes significant for wires greater in diameter than about an inch. Because of the skin effect in part, multiple wires arranged in a circular pattern and separated by polymer spacers are often used in high capacity high voltage AC transmission lines. Thus, overhead HVDC powerlines can transport significantly more power for greater distances than AC lines, for two main reasons: the effective voltage can be higher, and the wires can be bigger. But DC lines were not developed initially to be capable of higher voltage, nor to be able to move more power than AC lines, but rather to make it possible to put high capacity power lines underground (for security) or under the ocean (to bring power to islands initially).
To understand why undergrounding HVDC lines for great distances is feasible, while undergrounding HVAC lines for more than about 40 miles is not, it is necessary to consider the capacitance of air-insulated overhead lines versus cables, which are typically surrounded by polymer insulation and soil. Capacitance is a property of every electrical circuit, not just capacitors (which are designed deliberately for high capacitance). A wire suspended in air has much less capacitance (by about a factor of 50-100) compared to a cable, in which the wire is surrounded both by polymeric insulation and soil. The capacitance limits how fast the voltage responds at the far end of a power line when voltage is applied at the near end. Capacitance has only a small transient effect on a DC power transmission line, delaying the voltage rise at the far end of the line by milliseconds at most when voltage is applied at the near end. When capacitance of an AC line is too high though, it has a quite dramatic effect; this is the case because at 60 Hz, the voltage reverses 120 times per second (8.33 milliseconds for per reversal); each time this happens, the “line capacitor” needs to be charged up before any power can flow through the line. The much higher capacitance of a cable (especially one that is located underground or undersea) means that this limiting line capacitance is reached for a much shorter cable (50 to 100 times shorter) than an overhead line. Thus at most short bits of an AC power transmission line can be placed underground, whereas there is no problem in terms of power flow with putting a DC power line underground.
Another important property that differentiates AC from DC power lines is that for an AC line, the line power must be synchronized with the local AC grid at both ends of the line, whereas DC power can bridge between two different synchronized AC grids that are not synchronized with each other. For this reason, DC power lines are often referred to as “asynchronous links” by power engineers. Examples where this is important involve power links between the Quebec AC grid and the Eastern US grid; between the Eastern and Western US grids; between the Texas grid (ERCOT) and everywhere else; and between the incompatible 50 Hz and 60 Hz regions in Japan .
Nearly all of the above factors would seem to favor DC over AC transmission, so why are most transmission lines, and virtually all power distribution lines AC? Simply put: transformers (which change voltage of electrical power) and circuit breakers are dramatically less expensive for AC than for DC power. At the time that the first long transmission lines were built by Westinghouse between Niagara Falls and New York City, there was no such thing as a DC/DC transformer, and that hard technical limitation persisted for a hundred years (which is why we have a strictly AC grid). Today, electronic DC/DC voltage transformers are found on every computer motherboard, and can be built for high voltage, high power conversion as well…but these devices are a lot more expensive at present than conventional transformers. However, being electronic devices, these DC/DC transformers have been on a steeply declining cost curve for some time now, and it is probable that they will in the future reach cost parity with conventional AC/AC transformers. This could mean we will have a DC grid in 100 years or so, but don’t hold your breath. Meanwhile DC circuit breakers are also a huge problem, especially at high power levels above one megawatt (MW). ABB recently announced a breakthrough on HVDC circuit breakers that they say will allow HVDC circuit breakers up to one gigawatt (GW; equal to 1000 MW; still well below what will be needed to implement a supergrid). ABB has not published either a cost for their new breaker (which I estimate will be about 100 times as high as comparable AC circuit breakers), nor on-state power loss figures (which I estimate will be ~0.25% of transmitted power). We still have a way to go to having a DC circuit breaker that is capable of enabling a supergrid, in spite of ABB’s efforts to convince us otherwise. This is a problem I have been working on; I call my solution a Ballistic Breaker™.
- distributed-energy
Get Published - Build a Following
The Energy Central Power Industry Network® is based on one core idea - power industry professionals helping each other and advancing the industry by sharing and learning from each other.
If you have an experience or insight to share or have learned something from a conference or seminar, your peers and colleagues on Energy Central want to hear about it. It's also easy to share a link to an article you've liked or an industry resource that you think would be helpful.
Start a Post » Learn more about posting on Energy Central »
- Start a Post
Electric System Operator City of Tallahassee Tallahassee, Florida
Senior Energy Market Analyst Denton Municipal Electric Denton, Texas
Analyst, Wholesale Energy Services, Mid-Continent Customized Energy Solutions Mid-Continent States, Remote
Chief Executive Officer Baldwin EMC Summerdale, Alabama
Director of Strategy and Performance Management Long Island Power Authority Uniondale, New York
Director of Grants Management Long Island Power Authority Uniondale, New York
Hydro Plant Compliance Electrical Engineer (III, Senior I, Senior II, Senior III) Chelan Public Utility District Wenatchee, Washington
Sponsors & Partners


DC to AC Power: Why is AC Used Instead of DC?
by A.J. | Apr 13, 2016 | EXELTECH News
Two types of electrical current can be used to power electrical equipment: Direct Current (DC), which is characterized by the unidirectional flow of an electrical charge; and Alternating Current (AC), which is characterized by an electrical charge that can reverse directions. In Thomas Edison’s day, the power grid delivered DC to businesses and residences, whereas today, the worldwide power grid is largely designed to deliver AC.
Why Did the Changeover Occur?
Initially, the main reason for switching the power grid from DC to AC power was that DC couldn’t travel long distances across power lines without degrading in intensity. This meant that an electricity generation station was needed to serve a relatively small number of users, which ultimately meant that the DC operating system contained far more power stations than would have been necessary had an AC operating system been in place.
With the invention of electricity transformers that could be implemented in the power grid, AC became the prefered form of electrical current, not only because it could travel long distance across power lines without degrading, but also because it eliminated the corollary problem of power users receiving increasingly weaker current the farther they were located away from a DC power hub. If you lived in a house located at the end of a DC power line, your lights would burn dimmer than lights in a residence that was located near the beginning of the line.
Edison Vs. Westinghouse
Despite the obvious financial and functional drawbacks of using a DC electricity system, Edison remained stringent in his belief that DC was the most preferred current, even in the face of the clear advantages that George Westinghouse’s AC electricity system offered. Westinghouse eventually won out, with his transition of DC to AC power ironically winning him the American Institute of Electrical Engineers (AIEE) Edison Medal “for meritorious achievement in connection with the development of the alternating current system.”
When the power grid switched from DC to AC power, manufacturers of electrical equipment naturally began developing their products to receive AC instead of DC, creating a permanent shift in how the equipment was designed that still exists today. Although using DC electricity in the power grid still has some proponents, the design of AC-based electrical equipment all but ensures that AC will be used in our electricity system for the foreseeable future.
Exeltech is manufacturer of industrial grade power inverters and power converters that come with the certifications that companies and organizations need to address their unique electricity conduction requirements. For 25 years, we’ve supplied stock equipment and custom equipment to numerous entities in various industries. To inquire about our products, please call us today at (800) 886-4683, or use the contact form on our website.
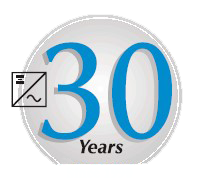
Excellence in Manufacturing Through American Pride
Made in the USA

Call Us Toll Free
800-866-4683.

I Want a FREE Quote!
Email Address
Phone Number
Company Name
Exeltech Series/Part Number
DC Input Voltage
Total Power (Watts)
Additional Specifications

The Most Trusted Provider of Inverters Worldwide!
- Privacy Policy
Headquarters
7317 Jack Newell Blvd N Fort Worth, TX 76118 Phone: 800-886-4683 Phone: 817-595-4969 Fax: 817-595-1290
Why is it that AC current can be transported over long distances whereas dc current can't be done so?
What's good for long-distance power transmission is high voltages. the higher the voltage, the lower the resistive losses in the cable. that's why mains power is transmitted at many hundreds of thousands of volts, and is only stepped down to the 110v or 230v that you're used to when it is near your building. ac is much easier (therefore cheaper) to step up and down in voltage than dc using a transformer, and can be done more efficiently (typcially, currently) than the much more expensive and complex dc step-up/step-down systems. that is why ac is preferred for mains power transmission over dc..

How ac can travel large distances whereas dc cannot


IMAGES
VIDEO
COMMENTS
Skin effect experienced with AC. There is no skin effect with DC. Higher voltage allowed with DC for the same transmission lines. The lines have to withstand the peak voltage. With AC, that is 1.4 times higher than the RMS. With DC, the RMS and peak voltages are the same. However, the power transmitted is the current times the RMS, not peak ...
skatastic57. • 11 yr. ago. From a technical perspective your question assumes incorrectly that AC is better. DC is actually better for traveling over long distances. The reason DC isn't used extensively is because it is not easy to change the voltage of DC. It is also tough to switch from DC to AC. One example of a HVDC (high volt DC) line ...
With ac the swing in the voltage is much higher than the dc voltage carting the same amount of power. So a $\rm 1MV$ rms ac power line has a voltage swing of $\pm \rm 1.4 MV$ and so with an ac power line the insulation has be able to withstand voltages which are 1.4 times than dc power lines. You might imagine this is a constraint for high ...
We now know that for either AC or DC, long-distance power lines must transmit power at low current and at very high voltages because of the power wasted by either modus in high current transmission. But, as illustrated below, at long distances even at 400,000 volts, AC power lines, still waste far more power than do DC power lines.
Direct current is a bit easier to understand than alternating current. Rather than oscillating back and forth, DC provides a constant voltage or current. Generating DC. DC can be generated in a number of ways: An AC generator equipped with a device called a "commutator" can produce direct current; Use of a device called a "rectifier" that ...
The current (electric charge) only flows in one direction in case of DC (direct current). But in AC (Alternating current) electric charge changes direction periodically. Not only current but also the voltage reverses because of the change in the current flow. The AC versus DC debate personifies the War of Curre nts, as it is called today, in ...
DC is constant and moves in one direction. "A simple way to visualize the difference is that, when graphed, a DC current looks like a flat line, whereas the flow of AC on a graph makes a sinusoid or wave-like pattern," says Berggren. "This is because AC changes over time in an oscillating repetition — the up curve indicates the current ...
Does AC in Long-Distance Transmission Travel Further Than DC? As discussed in the previous section, it is not true that AC travels farther than DC; it is all because AC can be transmitted with less power loss. Resistance in the transmission cables and wires is the same, and heat is produced by the current flow in them. ...
Direct current (DC) is the flow of electric charge in only one direction. It is the steady state of a constant-voltage circuit. Most well-known applications, however, use a time-varying voltage source. Alternating current (AC) is the flow of electric charge that periodically reverses direction. If the source varies periodically, particularly ...
A battery is more effective and also doesn't have skips in the power supply that an AC has since its voltage is sinusoidal. Also, using AC requires a transformer when transferring power of large distances. Also a DC does not introduce reactance in the line which transfers to higher power transfer in the line. This leads to lower cost.
Starting in the late 1880s, Thomas Edison and Nikola Tesla were embroiled in a battle now known as the War of the Currents. Edison developed direct current -- current that runs continually in a single direction, like in a battery or a fuel cell. During the early years of electricity, direct current (shorthanded as DC) was the standard in the U.S.
Other advantages of AC include: [5] Low maintenance costs of high speed AC motors. Easy to interrupt the current (ie. with a circuit breaker) due to the current going to zero naturally every 1/2 cycle. For example, a circuit breaker can interrupt about 1/20th as much DC as AC current.
AC does not "travel further" than DC. On the contrary the skin effect you're referring means a DC cable can carry a larger current than AC with the same heating losses. DC also doesn't suffer from losses due to capacitance and dielectric like AC does. As DaffGrind says : The reason AC is used and is more practical is that you can easily ...
DC power does not entail the phase factor. Unlike AC, DC can be stored. DC systems generally incur fewer losses, and the power transmitted is high. Cons. DC systems are more expensive due to higher insulation requirements. Unlike AC, the level of DC voltage cannot be changed easily without losing considerable energy.
Wire diameter is limited for AC transmission lines due to the " skin effect " that prevents an AC current from penetrating to the center of a large wire, whereas a DC line can be arbitrarily thick. At 60 Hz, the skin effect becomes significant for wires greater in diameter than about an inch.
At 500V, 1MW would be roughly 2,000 Amps. Ohms law shows that the voltage drop across the transmission line is 2, 000A × 0.1Ω = 200V 2, 000 A × 0.1 Ω = 200 V. This means that 40% of our power is lost in the process of sending it, and our voltage at the other side is a lousy 300V. At 500kV, our current is only 2 Amps.
Power is proportional to the square of the voltage. The same transmission line can carry twice the power at a steady DC voltage that is the same as the peak AC voltage. Skin effect. With AC, the outer edges of the cable carry more of the current. This makes the cable appear to have a higher resistance at AC than it does at DC.
When the power grid switched from DC to AC power, manufacturers of electrical equipment naturally began developing their products to receive AC instead of DC, creating a permanent shift in how the equipment was designed that still exists today. Although using DC electricity in the power grid still has some proponents, the design of AC-based ...
The waves created in the alternator are significant for AC power's use. The wave-like motion of AC power gives this electricity form an advantage over DC power. Because it moves in waves, this electricity format can travel farther than DC power. Most outlets in buildings provide AC power.
Yes. DC current will travel through the entirety of the wire -- AC current will tend to move on the outside of the conductor (see Skin Effect). This will cause slightly more ohmic heating in AC than in DC. That's why AC wire is usually stranded while DC wire is usually solid -- more strands = more surface area.
AC is much easier (therefore cheaper) to step up and down in voltage than DC using a transformer, and can be done more efficiently (typcially, currently) than the much more expensive and complex DC step-up/step-down systems. That is why AC is preferred for mains power transmission over DC. ... How ac can travel large distances whereas dc cannot. Q.