Travelling Wave
When something about the physical world changes, the information about that disturbance gradually moves outwards, away from the source, in every direction. As the information travels, it travels in the form of a wave. Sound to our ears, light to our eyes, and electromagnetic radiation to our mobile phones are all transported in the form of waves. A good visual example of the propagation of waves is the waves created on the surface of the water when a stone is dropped into a lake. In this article, we will be learning more about travelling waves.

Describing a Wave
A wave can be described as a disturbance in a medium that travels transferring momentum and energy without any net motion of the medium. A wave in which the positions of maximum and minimum amplitude travel through the medium is known as a travelling wave. To better understand a wave, let us think of the disturbance caused when we jump on a trampoline. When we jump on a trampoline, the downward push that we create at a point on the trampoline slightly moves the material next to it downward too.
When the created disturbance travels outward, the point at which our feet first hit the trampoline recovers moving outward because of the tension force in the trampoline and that moves the surrounding nearby materials outward too. This up and down motion gradually ripples out as it covers more area of the trampoline. And, this disturbance takes the shape of a wave.
Following are a few important points to remember about the wave:
- The high points in the wave are known as crests and the low points in the wave are known as troughs.
- The maximum distance of the disturbance of the wave from the mid-point to either the top of the crest or to the bottom of a trough is known as amplitude.
- The distance between two adjacent crests or two adjacent troughs is known as a wavelength and is denoted by 𝛌.
- The time interval of one complete vibration is known as a time period.
- The number of vibrations the wave undergoes in one second is known as a frequency.
- The relationship between the time period and frequency is given as follows:
- The speed of a wave is given by the equation
Different Types of Waves
Different types of waves exhibit distinct characteristics. These characteristics help us distinguish between wave types. The orientation of particle motion relative to the direction of wave propagation is one way the traveling waves are distinguished. Following are the different types of waves categorized based on the particle motion:
- Pulse Waves – A pulse wave is a wave comprising only one disturbance or only one crest that travels through the transmission medium.
- Continuous Waves – A continuous-wave is a waveform of constant amplitude and frequency.
- Transverse Waves – In a transverse wave, the motion of the particle is perpendicular to the direction of propagation of the wave.
- Longitudinal Waves – Longitudinal waves are the waves in which the motion of the particle is in the same direction as the propagation of the wave.
Although they are different, there is one property common between them and that is the transportation of energy. An object in simple harmonic motion has an energy of
Constructive and Destructive Interference
A phenomenon in which two waves superimpose to form a resultant wave of lower, greater, or the same amplitude is known as interference. Constructive and destructive interference occurs due to the interaction of waves that are correlated with each other either because of the same frequency or because they come from the same source. The interference effects can be observed in all types of waves such as gravity waves and light waves.
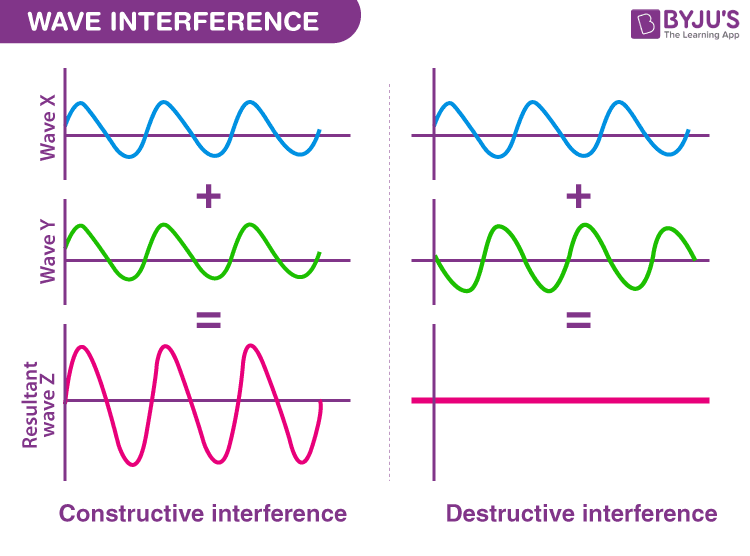
According to the principle of superposition of the waves , when two or more propagating waves of the same type are incidents on the same point, the resultant amplitude is equal to the vector sum of the amplitudes of the individual waves. When a crest of a wave meets a crest of another wave of the same frequency at the same point, then the resultant amplitude is the sum of the individual amplitudes. This type of interference is known as constructive interference. If a crest of a wave meets a trough of another wave, then the resulting amplitude is equal to the difference in the individual amplitudes and this is known as destructive interference.
Stay tuned to BYJU’S to learn more physics concepts with the help of interactive videos.
Watch the video and understand longitudinal and transverse waves in detail.

Frequently Asked Questions – FAQs
What is a pulse wave, what are longitudinal waves, what is superposition of waves, what is electromagnetic radiation, what is constructive interference.
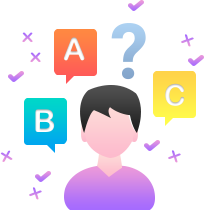
Put your understanding of this concept to test by answering a few MCQs. Click ‘Start Quiz’ to begin!
Select the correct answer and click on the “Finish” button Check your score and answers at the end of the quiz
Visit BYJU’S for all Physics related queries and study materials
Your result is as below
Request OTP on Voice Call
Leave a Comment Cancel reply
Your Mobile number and Email id will not be published. Required fields are marked *
Post My Comment

- Share Share
Register with BYJU'S & Download Free PDFs
Register with byju's & watch live videos.

Revision notes for IB Physics
Topic 4: waves.
See the guide for this topic.
4.1 – Oscillations
Simple harmonic oscillations.
Oscillations are periodic motions which center around an equilibrium position.
Simple harmonic motion (SHM) is a special type of oscillation. For example:
- The simple pendulum
- The vibration of strings in a violin
- The spring-mass system, where the mass is initially displaced to produce a periodic motion around the equilibrium position
An object undergoes SHM if it experiences a force which is proportional and opposite of the displacement from its equilibrium position.
for a pendulum
for a spring-mass system
Time period, frequency, amplitude, displacement and phase difference

Conditions for simple harmonic motion
- When the body is displaced from equilibrium, there must exist a restoring force (a force that wants to pull the body back to equilibrium).
- The magnitude of the restoring force must be proportional to the displacement of the body and acts towards the equilibrium.
4.2 – Travelling waves
Travelling waves.
A travelling wave is a continuous disturbance in a medium characterized by repeating oscillations. For example:
- A rope that is flicked up and down continuously creates a repeating disturbance similar to the shape of a sine/cosine wave.
Energy is transferred by waves.
Matter is not transferred by waves.
The direction of a wave is defined by the direction of the energy transfer.
Wavelength, frequency, period and wave speed
Wavelength, frequency, and period follow the same rules of SHM.
Wave speed can be calculated by the following equation

Transverse and longitudinal waves
The nature of electromagnetic waves.
All EM waves travel in vacuum at the same speed of 3*10^8m/s.
EM waves are transverse waves.

The nature of sound waves
The speed of sound in 20 degrees Celsius dry air is approximately 343.2m/s.
Sound waves are longitudinal waves.
4.3 – Wave characteristics
Wavefronts and rays.
Wavefronts:
- Lines joining points which vibrate in phase.
- Can be straight lines or curves.
- The distance between successive wavefronts is the wavelength of the wave.
- Lines which indicate the direction of wave propagation.
- Rays are perpendicular to wavefronts.

Amplitude and intensity
The amplitude and intensity of a wave depends on its energy.
The intensity of a wave is proportional to the square of its amplitude (I∝A^2).

See previous section with the same title.
Superposition
The principle of superposition states that the net displacement of the underlying medium for a wave is equal to the sum of the individual wave displacements.

The left shows constructive interference (superposition) where the two waves add up (e.g. 1+1=2). The right shows deconstructive interference (superposition) where the two waves cancel each other (e.g. 1+(-1)=0).
Polarization
Light is a transverse wave (polarization only occur to transverse waves).
The polarization of light refers to the orientation of the oscillation in the underlying electric field.
Light is plane polarized if the electric field oscillates in one plane.

Left shows unpolarized light and right shows polarized light.
Polarization by reflection
When light is transmitted across a boundary between two mediums with different refractive indexes, part of the light is reflected and the remaining part is refracted (for further explanation, see section 4.4).
The light reflected is partially polarized, meaning that it is a mixture of polarized light and unpolarized light.
The extent to which the reflected light is polarized depends on the angle of incidence and the refractive index of the two mediums.
The angle of incidence at which the reflected light is totally polarized is called the Brewster’s angle (ϕ) given by the equation
where n1 and n2 are the refractive indexes for their respective mediums
When the angle of incidence is equal to Brewster’s angle, the reflected ray is totally polarized and the reflected ray is perpendicular to the refracted ray.

Polarizers and Analyzers
- A polarizer is a sheet of material which polarizes light.
- When unpolarized light passes through a polarizer, its intensity is reduced by 50%.
- When polarized light passes through a polarizer, its intensity will be reduced by a factor dependent on the orientation of the polarizer. This property allows us to deduce the polarization of light by using a polarizer.
- A polarizer used for this purpose is called an analyzer.
Malus’ Law relates the incident intensity and transmitted intensity of light passing through a polarizer and an analyzer.

where I is the transmitted intensity, I0 is the initial light intensity upon the analyzer, θ is the angle between the transmission axis and the analyzer.
When light passes through an optically active substance, the plane of polarization rotates.
4.4 – Wave behaviour
Reflection and refraction.
Angle of incidence = Angle of reflection
Reflection of waves from a fixed end is inverted.
Reflection of waves from a free end is not inverted.
Refraction is the change in direction of a wave when it transmits from one medium to another.

The angle of incidence and the angle of refraction can be determined by Snell’s law given by the following formula

Fast-to-slow: towards normal; slow-to-fast: away from normal
In addition, the refractive index n1 and n2 are related by the following equation

where v1 and v2 are the speed of the waves in their respective mediums and λ1 and λ2 are the wavelength of the waves of their respective mediums
Snell’s law, critical angle and total internal reflection
See previous section (Reflection and refraction) for Snell’s law.

Diffraction through a single-slit and around objects
- Diffraction through a single-slit
Single-slit equations are not required for the standard level course.
- Diffraction around objects
Interference patterns
Maximums form at constructive interference (the maximum is shown by 1-2) and minimums form at deconstructive interference (the first minimum is shown by 3-4).

Double-slit interference
Like single-slit diffraction, double-slit diffraction occurs via the same methods of interference and has a similar diffraction pattern.

Path difference

4.5 – Standing waves
The nature of standing waves.
Standing waves (stationary) waves result from the superposition of two opposite waves which are otherwise identical.
Energy is not transferred by standing waves.
A wave hits a wall and is reflected identically opposite.
The black wave shows the wave created by the superposition of the blue and green waves.
Boundary conditions
Air particles can oscillate and create standing waves in pipes with open or closed ends.
Antinodes are positioned at open ends and nodes are positioned at closed ends.
Standing waves on a string is equivalent to that in a pipe which is closed on both ends (nodes-node).

The following table summarizes the behavior of standing waves in pipes and strings:
Nodes and antinodes
Positions along the wave which are fixed are called nodes (minimum) and those with the largest displacement are called antinodes (maximum).
For standing waves, the distance between adjacent nodes = the distance between adjacent antinodes = λ/2.
Difference between standing waves and travelling waves
Share this:
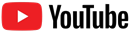
- TPC and eLearning
- What's NEW at TPC?
- Read Watch Interact
- Practice Review Test
- Teacher-Tools
- Subscription Selection
- Seat Calculator
- Ad Free Account
- Edit Profile Settings
- Classes (Version 2)
- Student Progress Edit
- Task Properties
- Export Student Progress
- Task, Activities, and Scores
- Metric Conversions Questions
- Metric System Questions
- Metric Estimation Questions
- Significant Digits Questions
- Proportional Reasoning
- Acceleration
- Distance-Displacement
- Dots and Graphs
- Graph That Motion
- Match That Graph
- Name That Motion
- Motion Diagrams
- Pos'n Time Graphs Numerical
- Pos'n Time Graphs Conceptual
- Up And Down - Questions
- Balanced vs. Unbalanced Forces
- Change of State
- Force and Motion
- Mass and Weight
- Match That Free-Body Diagram
- Net Force (and Acceleration) Ranking Tasks
- Newton's Second Law
- Normal Force Card Sort
- Recognizing Forces
- Air Resistance and Skydiving
- Solve It! with Newton's Second Law
- Which One Doesn't Belong?
- Component Addition Questions
- Head-to-Tail Vector Addition
- Projectile Mathematics
- Trajectory - Angle Launched Projectiles
- Trajectory - Horizontally Launched Projectiles
- Vector Addition
- Vector Direction
- Which One Doesn't Belong? Projectile Motion
- Forces in 2-Dimensions
- Being Impulsive About Momentum
- Explosions - Law Breakers
- Hit and Stick Collisions - Law Breakers
- Case Studies: Impulse and Force
- Impulse-Momentum Change Table
- Keeping Track of Momentum - Hit and Stick
- Keeping Track of Momentum - Hit and Bounce
- What's Up (and Down) with KE and PE?
- Energy Conservation Questions
- Energy Dissipation Questions
- Energy Ranking Tasks
- LOL Charts (a.k.a., Energy Bar Charts)
- Match That Bar Chart
- Words and Charts Questions
- Name That Energy
- Stepping Up with PE and KE Questions
- Case Studies - Circular Motion
- Circular Logic
- Forces and Free-Body Diagrams in Circular Motion
- Gravitational Field Strength
- Universal Gravitation
- Angular Position and Displacement
- Linear and Angular Velocity
- Angular Acceleration
- Rotational Inertia
- Balanced vs. Unbalanced Torques
- Getting a Handle on Torque
- Torque-ing About Rotation
- Properties of Matter
- Fluid Pressure
- Buoyant Force
- Sinking, Floating, and Hanging
- Pascal's Principle
- Flow Velocity
- Bernoulli's Principle
- Balloon Interactions
- Charge and Charging
- Charge Interactions
- Charging by Induction
- Conductors and Insulators
- Coulombs Law
- Electric Field
- Electric Field Intensity
- Polarization
- Case Studies: Electric Power
- Know Your Potential
- Light Bulb Anatomy
- I = ∆V/R Equations as a Guide to Thinking
- Parallel Circuits - ∆V = I•R Calculations
- Resistance Ranking Tasks
- Series Circuits - ∆V = I•R Calculations
- Series vs. Parallel Circuits
- Equivalent Resistance
- Period and Frequency of a Pendulum
- Pendulum Motion: Velocity and Force
- Energy of a Pendulum
- Period and Frequency of a Mass on a Spring
- Horizontal Springs: Velocity and Force
- Vertical Springs: Velocity and Force
- Energy of a Mass on a Spring
- Decibel Scale
- Frequency and Period
- Closed-End Air Columns
- Name That Harmonic: Strings
- Rocking the Boat
- Wave Basics
- Matching Pairs: Wave Characteristics
- Wave Interference
- Waves - Case Studies
- Color Addition and Subtraction
- Color Filters
- If This, Then That: Color Subtraction
- Light Intensity
- Color Pigments
- Converging Lenses
- Curved Mirror Images
- Law of Reflection
- Refraction and Lenses
- Total Internal Reflection
- Who Can See Who?
- Formulas and Atom Counting
- Atomic Models
- Bond Polarity
- Entropy Questions
- Cell Voltage Questions
- Heat of Formation Questions
- Reduction Potential Questions
- Oxidation States Questions
- Measuring the Quantity of Heat
- Hess's Law
- Oxidation-Reduction Questions
- Galvanic Cells Questions
- Thermal Stoichiometry
- Molecular Polarity
- Quantum Mechanics
- Balancing Chemical Equations
- Bronsted-Lowry Model of Acids and Bases
- Classification of Matter
- Collision Model of Reaction Rates
- Density Ranking Tasks
- Dissociation Reactions
- Complete Electron Configurations
- Elemental Measures
- Enthalpy Change Questions
- Equilibrium Concept
- Equilibrium Constant Expression
- Equilibrium Calculations - Questions
- Equilibrium ICE Table
- Intermolecular Forces Questions
- Ionic Bonding
- Lewis Electron Dot Structures
- Limiting Reactants
- Line Spectra Questions
- Mass Stoichiometry
- Measurement and Numbers
- Metals, Nonmetals, and Metalloids
- Metric Estimations
- Metric System
- Molarity Ranking Tasks
- Mole Conversions
- Name That Element
- Names to Formulas
- Names to Formulas 2
- Nuclear Decay
- Particles, Words, and Formulas
- Periodic Trends
- Precipitation Reactions and Net Ionic Equations
- Pressure Concepts
- Pressure-Temperature Gas Law
- Pressure-Volume Gas Law
- Chemical Reaction Types
- Significant Digits and Measurement
- States Of Matter Exercise
- Stoichiometry Law Breakers
- Stoichiometry - Math Relationships
- Subatomic Particles
- Spontaneity and Driving Forces
- Gibbs Free Energy
- Volume-Temperature Gas Law
- Acid-Base Properties
- Energy and Chemical Reactions
- Chemical and Physical Properties
- Valence Shell Electron Pair Repulsion Theory
- Writing Balanced Chemical Equations
- Mission CG1
- Mission CG10
- Mission CG2
- Mission CG3
- Mission CG4
- Mission CG5
- Mission CG6
- Mission CG7
- Mission CG8
- Mission CG9
- Mission EC1
- Mission EC10
- Mission EC11
- Mission EC12
- Mission EC2
- Mission EC3
- Mission EC4
- Mission EC5
- Mission EC6
- Mission EC7
- Mission EC8
- Mission EC9
- Mission RL1
- Mission RL2
- Mission RL3
- Mission RL4
- Mission RL5
- Mission RL6
- Mission KG7
- Mission RL8
- Mission KG9
- Mission RL10
- Mission RL11
- Mission RM1
- Mission RM2
- Mission RM3
- Mission RM4
- Mission RM5
- Mission RM6
- Mission RM8
- Mission RM10
- Mission LC1
- Mission RM11
- Mission LC2
- Mission LC3
- Mission LC4
- Mission LC5
- Mission LC6
- Mission LC8
- Mission SM1
- Mission SM2
- Mission SM3
- Mission SM4
- Mission SM5
- Mission SM6
- Mission SM8
- Mission SM10
- Mission KG10
- Mission SM11
- Mission KG2
- Mission KG3
- Mission KG4
- Mission KG5
- Mission KG6
- Mission KG8
- Mission KG11
- Mission F2D1
- Mission F2D2
- Mission F2D3
- Mission F2D4
- Mission F2D5
- Mission F2D6
- Mission KC1
- Mission KC2
- Mission KC3
- Mission KC4
- Mission KC5
- Mission KC6
- Mission KC7
- Mission KC8
- Mission AAA
- Mission SM9
- Mission LC7
- Mission LC9
- Mission NL1
- Mission NL2
- Mission NL3
- Mission NL4
- Mission NL5
- Mission NL6
- Mission NL7
- Mission NL8
- Mission NL9
- Mission NL10
- Mission NL11
- Mission NL12
- Mission MC1
- Mission MC10
- Mission MC2
- Mission MC3
- Mission MC4
- Mission MC5
- Mission MC6
- Mission MC7
- Mission MC8
- Mission MC9
- Mission RM7
- Mission RM9
- Mission RL7
- Mission RL9
- Mission SM7
- Mission SE1
- Mission SE10
- Mission SE11
- Mission SE12
- Mission SE2
- Mission SE3
- Mission SE4
- Mission SE5
- Mission SE6
- Mission SE7
- Mission SE8
- Mission SE9
- Mission VP1
- Mission VP10
- Mission VP2
- Mission VP3
- Mission VP4
- Mission VP5
- Mission VP6
- Mission VP7
- Mission VP8
- Mission VP9
- Mission WM1
- Mission WM2
- Mission WM3
- Mission WM4
- Mission WM5
- Mission WM6
- Mission WM7
- Mission WM8
- Mission WE1
- Mission WE10
- Mission WE2
- Mission WE3
- Mission WE4
- Mission WE5
- Mission WE6
- Mission WE7
- Mission WE8
- Mission WE9
- Vector Walk Interactive
- Name That Motion Interactive
- Kinematic Graphing 1 Concept Checker
- Kinematic Graphing 2 Concept Checker
- Graph That Motion Interactive
- Two Stage Rocket Interactive
- Rocket Sled Concept Checker
- Force Concept Checker
- Free-Body Diagrams Concept Checker
- Free-Body Diagrams The Sequel Concept Checker
- Skydiving Concept Checker
- Elevator Ride Concept Checker
- Vector Addition Concept Checker
- Vector Walk in Two Dimensions Interactive
- Name That Vector Interactive
- River Boat Simulator Concept Checker
- Projectile Simulator 2 Concept Checker
- Projectile Simulator 3 Concept Checker
- Hit the Target Interactive
- Turd the Target 1 Interactive
- Turd the Target 2 Interactive
- Balance It Interactive
- Go For The Gold Interactive
- Egg Drop Concept Checker
- Fish Catch Concept Checker
- Exploding Carts Concept Checker
- Collision Carts - Inelastic Collisions Concept Checker
- Its All Uphill Concept Checker
- Stopping Distance Concept Checker
- Chart That Motion Interactive
- Roller Coaster Model Concept Checker
- Uniform Circular Motion Concept Checker
- Horizontal Circle Simulation Concept Checker
- Vertical Circle Simulation Concept Checker
- Race Track Concept Checker
- Gravitational Fields Concept Checker
- Orbital Motion Concept Checker
- Angular Acceleration Concept Checker
- Balance Beam Concept Checker
- Torque Balancer Concept Checker
- Aluminum Can Polarization Concept Checker
- Charging Concept Checker
- Name That Charge Simulation
- Coulomb's Law Concept Checker
- Electric Field Lines Concept Checker
- Put the Charge in the Goal Concept Checker
- Circuit Builder Concept Checker (Series Circuits)
- Circuit Builder Concept Checker (Parallel Circuits)
- Circuit Builder Concept Checker (∆V-I-R)
- Circuit Builder Concept Checker (Voltage Drop)
- Equivalent Resistance Interactive
- Pendulum Motion Simulation Concept Checker
- Mass on a Spring Simulation Concept Checker
- Particle Wave Simulation Concept Checker
- Boundary Behavior Simulation Concept Checker
- Slinky Wave Simulator Concept Checker
- Simple Wave Simulator Concept Checker
- Wave Addition Simulation Concept Checker
- Standing Wave Maker Simulation Concept Checker
- Color Addition Concept Checker
- Painting With CMY Concept Checker
- Stage Lighting Concept Checker
- Filtering Away Concept Checker
- InterferencePatterns Concept Checker
- Young's Experiment Interactive
- Plane Mirror Images Interactive
- Who Can See Who Concept Checker
- Optics Bench (Mirrors) Concept Checker
- Name That Image (Mirrors) Interactive
- Refraction Concept Checker
- Total Internal Reflection Concept Checker
- Optics Bench (Lenses) Concept Checker
- Kinematics Preview
- Velocity Time Graphs Preview
- Moving Cart on an Inclined Plane Preview
- Stopping Distance Preview
- Cart, Bricks, and Bands Preview
- Fan Cart Study Preview
- Friction Preview
- Coffee Filter Lab Preview
- Friction, Speed, and Stopping Distance Preview
- Up and Down Preview
- Projectile Range Preview
- Ballistics Preview
- Juggling Preview
- Marshmallow Launcher Preview
- Air Bag Safety Preview
- Colliding Carts Preview
- Collisions Preview
- Engineering Safer Helmets Preview
- Push the Plow Preview
- Its All Uphill Preview
- Energy on an Incline Preview
- Modeling Roller Coasters Preview
- Hot Wheels Stopping Distance Preview
- Ball Bat Collision Preview
- Energy in Fields Preview
- Weightlessness Training Preview
- Roller Coaster Loops Preview
- Universal Gravitation Preview
- Keplers Laws Preview
- Kepler's Third Law Preview
- Charge Interactions Preview
- Sticky Tape Experiments Preview
- Wire Gauge Preview
- Voltage, Current, and Resistance Preview
- Light Bulb Resistance Preview
- Series and Parallel Circuits Preview
- Thermal Equilibrium Preview
- Linear Expansion Preview
- Heating Curves Preview
- Electricity and Magnetism - Part 1 Preview
- Electricity and Magnetism - Part 2 Preview
- Vibrating Mass on a Spring Preview
- Period of a Pendulum Preview
- Wave Speed Preview
- Slinky-Experiments Preview
- Standing Waves in a Rope Preview
- Sound as a Pressure Wave Preview
- DeciBel Scale Preview
- DeciBels, Phons, and Sones Preview
- Sound of Music Preview
- Shedding Light on Light Bulbs Preview
- Models of Light Preview
- Electromagnetic Radiation Preview
- Electromagnetic Spectrum Preview
- EM Wave Communication Preview
- Digitized Data Preview
- Light Intensity Preview
- Concave Mirrors Preview
- Object Image Relations Preview
- Snells Law Preview
- Reflection vs. Transmission Preview
- Magnification Lab Preview
- Reactivity Preview
- Ions and the Periodic Table Preview
- Periodic Trends Preview
- Intermolecular Forces Preview
- Melting Points and Boiling Points Preview
- Reaction Rates Preview
- Ammonia Factory Preview
- Stoichiometry Preview
- Nuclear Chemistry Preview
- Gaining Teacher Access
- Tasks and Classes
- Tasks - Classic
- Subscription
- Subscription Locator
- 1-D Kinematics
- Newton's Laws
- Vectors - Motion and Forces in Two Dimensions
- Momentum and Its Conservation
- Work and Energy
- Circular Motion and Satellite Motion
- Thermal Physics
- Static Electricity
- Electric Circuits
- Vibrations and Waves
- Sound Waves and Music
- Light and Color
- Reflection and Mirrors
- About the Physics Interactives
- Task Tracker
- Usage Policy
- Newtons Laws
- Vectors and Projectiles
- Forces in 2D
- Momentum and Collisions
- Circular and Satellite Motion
- Balance and Rotation
- Electromagnetism
- Waves and Sound
- Atomic Physics
- Forces in Two Dimensions
- Work, Energy, and Power
- Circular Motion and Gravitation
- Sound Waves
- 1-Dimensional Kinematics
- Circular, Satellite, and Rotational Motion
- Einstein's Theory of Special Relativity
- Waves, Sound and Light
- QuickTime Movies
- About the Concept Builders
- Pricing For Schools
- Directions for Version 2
- Measurement and Units
- Relationships and Graphs
- Rotation and Balance
- Vibrational Motion
- Reflection and Refraction
- Teacher Accounts
- Task Tracker Directions
- Kinematic Concepts
- Kinematic Graphing
- Wave Motion
- Sound and Music
- About CalcPad
- 1D Kinematics
- Vectors and Forces in 2D
- Simple Harmonic Motion
- Rotational Kinematics
- Rotation and Torque
- Rotational Dynamics
- Electric Fields, Potential, and Capacitance
- Transient RC Circuits
- Light Waves
- Units and Measurement
- Stoichiometry
- Molarity and Solutions
- Thermal Chemistry
- Acids and Bases
- Kinetics and Equilibrium
- Solution Equilibria
- Oxidation-Reduction
- Nuclear Chemistry
- NGSS Alignments
- 1D-Kinematics
- Projectiles
- Circular Motion
- Magnetism and Electromagnetism
- Graphing Practice
- About the ACT
- ACT Preparation
- For Teachers
- Other Resources
- Newton's Laws of Motion
- Work and Energy Packet
- Static Electricity Review
- Solutions Guide
- Solutions Guide Digital Download
- Motion in One Dimension
- Work, Energy and Power
- Algebra Based Physics
- TaskTracker
- Other Tools
- Frequently Asked Questions
- Purchasing the Download
- Purchasing the CD
- Purchasing the Digital Download
- About the NGSS Corner
- NGSS Search
- Force and Motion DCIs - High School
- Energy DCIs - High School
- Wave Applications DCIs - High School
- Force and Motion PEs - High School
- Energy PEs - High School
- Wave Applications PEs - High School
- Crosscutting Concepts
- The Practices
- Physics Topics
- NGSS Corner: Activity List
- NGSS Corner: Infographics
- About the Toolkits
- Position-Velocity-Acceleration
- Position-Time Graphs
- Velocity-Time Graphs
- Newton's First Law
- Newton's Second Law
- Newton's Third Law
- Terminal Velocity
- Projectile Motion
- Forces in 2 Dimensions
- Impulse and Momentum Change
- Momentum Conservation
- Work-Energy Fundamentals
- Work-Energy Relationship
- Roller Coaster Physics
- Satellite Motion
- Electric Fields
- Circuit Concepts
- Series Circuits
- Parallel Circuits
- Describing-Waves
- Wave Behavior Toolkit
- Standing Wave Patterns
- Resonating Air Columns
- Wave Model of Light
- Plane Mirrors
- Curved Mirrors
- Teacher Guide
- Using Lab Notebooks
- Current Electricity
- Light Waves and Color
- Reflection and Ray Model of Light
- Refraction and Ray Model of Light
- Classes (Legacy Version)
- Teacher Resources
- Subscriptions

- Newton's Laws
- Einstein's Theory of Special Relativity
- About Concept Checkers
- School Pricing
- Newton's Laws of Motion
- Newton's First Law
- Newton's Third Law
- Traveling Waves vs. Standing Waves
- Formation of Standing Waves
- Nodes and Anti-nodes
- Harmonics and Patterns
- Mathematics of Standing Waves
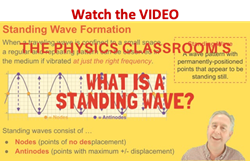
What is a Standing Wave Pattern?
It is however possible to have a wave confined to a given space in a medium and still produce a regular wave pattern that is readily discernible amidst the motion of the medium. For instance, if an elastic rope is held end-to-end and vibrated at just the right frequency , a wave pattern would be produced that assumes the shape of a sine wave and is seen to change over time. The wave pattern is only produced when one end of the rope is vibrated at just the right frequency. When the proper frequency is used, the interference of the incident wave and the reflected wave occur in such a manner that there are specific points along the medium that appear to be standing still. Because the observed wave pattern is characterized by points that appear to be standing still, the pattern is often called a standing wave pattern . There are other points along the medium whose displacement changes over time, but in a regular manner. These points vibrate back and forth from a positive displacement to a negative displacement; the vibrations occur at regular time intervals such that the motion of the medium is regular and repeating. A pattern is readily observable.
We Would Like to Suggest ...
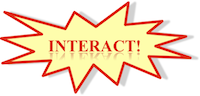
16.1 Traveling Waves
Learning objectives.
By the end of this section, you will be able to:
- Describe the basic characteristics of wave motion
- Define the terms wavelength, amplitude, period, frequency, and wave speed
- Explain the difference between longitudinal and transverse waves, and give examples of each type
- List the different types of waves
We saw in Oscillations that oscillatory motion is an important type of behavior that can be used to model a wide range of physical phenomena. Oscillatory motion is also important because oscillations can generate waves, which are of fundamental importance in physics. Many of the terms and equations we studied in the chapter on oscillations apply equally well to wave motion ( (Figure) ).
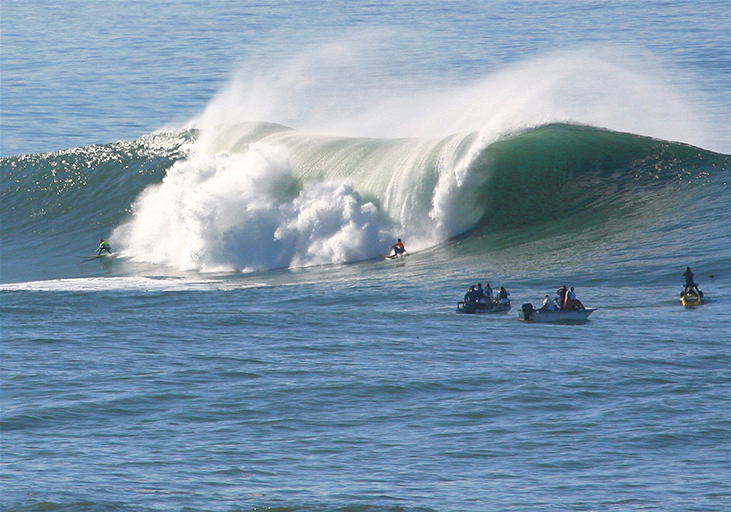
Figure 16.2 From the world of renewable energy sources comes the electric power-generating buoy. Although there are many versions, this one converts the up-and-down motion, as well as side-to-side motion, of the buoy into rotational motion in order to turn an electric generator, which stores the energy in batteries.
Types of Waves
A wave is a disturbance that propagates, or moves from the place it was created. There are three basic types of waves: mechanical waves, electromagnetic waves, and matter waves.
Basic mechanical waves are governed by Newton’s laws and require a medium. A medium is the substance a mechanical waves propagates through, and the medium produces an elastic restoring force when it is deformed. Mechanical waves transfer energy and momentum, without transferring mass. Some examples of mechanical waves are water waves, sound waves, and seismic waves. The medium for water waves is water; for sound waves, the medium is usually air. (Sound waves can travel in other media as well; we will look at that in more detail in Sound .) For surface water waves, the disturbance occurs on the surface of the water, perhaps created by a rock thrown into a pond or by a swimmer splashing the surface repeatedly. For sound waves, the disturbance is a change in air pressure, perhaps created by the oscillating cone inside a speaker or a vibrating tuning fork. In both cases, the disturbance is the oscillation of the molecules of the fluid. In mechanical waves, energy and momentum transfer with the motion of the wave, whereas the mass oscillates around an equilibrium point. (We discuss this in Energy and Power of a Wave .) Earthquakes generate seismic waves from several types of disturbances, including the disturbance of Earth’s surface and pressure disturbances under the surface. Seismic waves travel through the solids and liquids that form Earth. In this chapter, we focus on mechanical waves.
Electromagnetic waves are associated with oscillations in electric and magnetic fields and do not require a medium. Examples include gamma rays, X-rays, ultraviolet waves, visible light, infrared waves, microwaves, and radio waves. Electromagnetic waves can travel through a vacuum at the speed of light, [latex] v=c=2.99792458\,×\,{10}^{8}\,\text{m/s}. [/latex] For example, light from distant stars travels through the vacuum of space and reaches Earth. Electromagnetic waves have some characteristics that are similar to mechanical waves; they are covered in more detail in Electromagnetic Waves in volume 2 of this text.
Matter waves are a central part of the branch of physics known as quantum mechanics. These waves are associated with protons, electrons, neutrons, and other fundamental particles found in nature. The theory that all types of matter have wave-like properties was first proposed by Louis de Broglie in 1924. Matter waves are discussed in Photons and Matter Waves in the third volume of this text.
Mechanical Waves
Mechanical waves exhibit characteristics common to all waves, such as amplitude, wavelength, period, frequency, and energy. All wave characteristics can be described by a small set of underlying principles.
The simplest mechanical waves repeat themselves for several cycles and are associated with simple harmonic motion. These simple harmonic waves can be modeled using some combination of sine and cosine functions. For example, consider the simplified surface water wave that moves across the surface of water as illustrated in (Figure) . Unlike complex ocean waves, in surface water waves, the medium, in this case water, moves vertically, oscillating up and down, whereas the disturbance of the wave moves horizontally through the medium. In (Figure) , the waves causes a seagull to move up and down in simple harmonic motion as the wave crests and troughs (peaks and valleys) pass under the bird. The crest is the highest point of the wave, and the trough is the lowest part of the wave. The time for one complete oscillation of the up-and-down motion is the wave’s period T . The wave’s frequency is the number of waves that pass through a point per unit time and is equal to [latex] f=1\text{/}T. [/latex] The period can be expressed using any convenient unit of time but is usually measured in seconds; frequency is usually measured in hertz (Hz), where [latex] 1\,{\text{Hz}=1\,\text{s}}^{-1}. [/latex]
The length of the wave is called the wavelength and is represented by the Greek letter lambda [latex] (\lambda ) [/latex], which is measured in any convenient unit of length, such as a centimeter or meter. The wavelength can be measured between any two similar points along the medium that have the same height and the same slope. In (Figure) , the wavelength is shown measured between two crests. As stated above, the period of the wave is equal to the time for one oscillation, but it is also equal to the time for one wavelength to pass through a point along the wave’s path.
The amplitude of the wave ( A ) is a measure of the maximum displacement of the medium from its equilibrium position. In the figure, the equilibrium position is indicated by the dotted line, which is the height of the water if there were no waves moving through it. In this case, the wave is symmetrical, the crest of the wave is a distance [latex] \text{+}A [/latex] above the equilibrium position, and the trough is a distance [latex] \text{−}A [/latex] below the equilibrium position. The units for the amplitude can be centimeters or meters, or any convenient unit of distance.
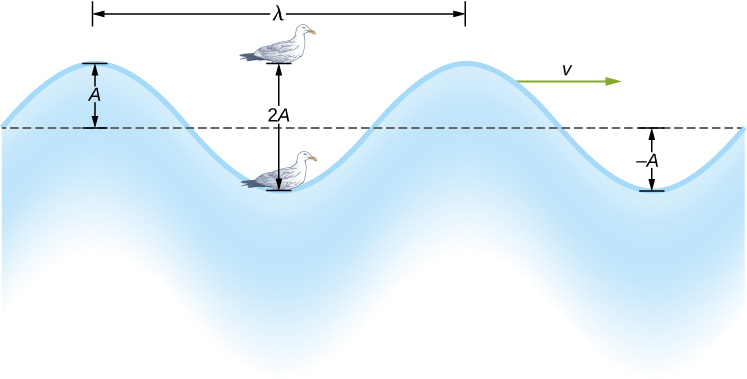
Figure 16.3 An idealized surface water wave passes under a seagull that bobs up and down in simple harmonic motion. The wave has a wavelength [latex] \lambda [/latex], which is the distance between adjacent identical parts of the wave. The amplitude A of the wave is the maximum displacement of the wave from the equilibrium position, which is indicated by the dotted line. In this example, the medium moves up and down, whereas the disturbance of the surface propagates parallel to the surface at a speed v.
The water wave in the figure moves through the medium with a propagation velocity [latex] \overset{\to }{v}. [/latex] The magnitude of the wave velocity is the distance the wave travels in a given time, which is one wavelength in the time of one period, and the wave speed is the magnitude of wave velocity. In equation form, this is
This fundamental relationship holds for all types of waves. For water waves, v is the speed of a surface wave; for sound, v is the speed of sound; and for visible light, v is the speed of light.
Transverse and Longitudinal Waves
We have seen that a simple mechanical wave consists of a periodic disturbance that propagates from one place to another through a medium. In (Figure) (a), the wave propagates in the horizontal direction, whereas the medium is disturbed in the vertical direction. Such a wave is called a transverse wave . In a transverse wave, the wave may propagate in any direction, but the disturbance of the medium is perpendicular to the direction of propagation. In contrast, in a longitudinal wave or compressional wave, the disturbance is parallel to the direction of propagation. (Figure) (b) shows an example of a longitudinal wave. The size of the disturbance is its amplitude A and is completely independent of the speed of propagation v .
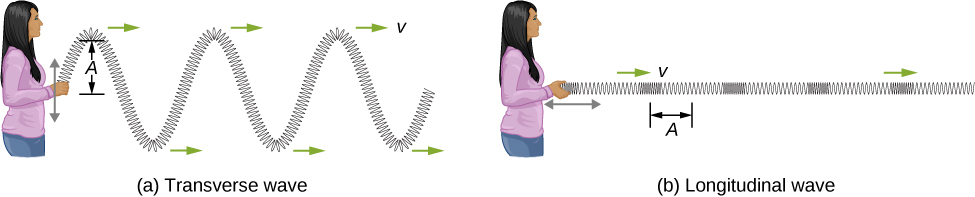
Figure 16.4 (a) In a transverse wave, the medium oscillates perpendicular to the wave velocity. Here, the spring moves vertically up and down, while the wave propagates horizontally to the right. (b) In a longitudinal wave, the medium oscillates parallel to the propagation of the wave. In this case, the spring oscillates back and forth, while the wave propagates to the right.
A simple graphical representation of a section of the spring shown in (Figure) (b) is shown in (Figure) . (Figure) (a) shows the equilibrium position of the spring before any waves move down it. A point on the spring is marked with a blue dot. (Figure) (b) through (g) show snapshots of the spring taken one-quarter of a period apart, sometime after the end of` the spring is oscillated back and forth in the x -direction at a constant frequency. The disturbance of the wave is seen as the compressions and the expansions of the spring. Note that the blue dot oscillates around its equilibrium position a distance A , as the longitudinal wave moves in the positive x -direction with a constant speed. The distance A is the amplitude of the wave. The y -position of the dot does not change as the wave moves through the spring. The wavelength of the wave is measured in part (d). The wavelength depends on the speed of the wave and the frequency of the driving force.
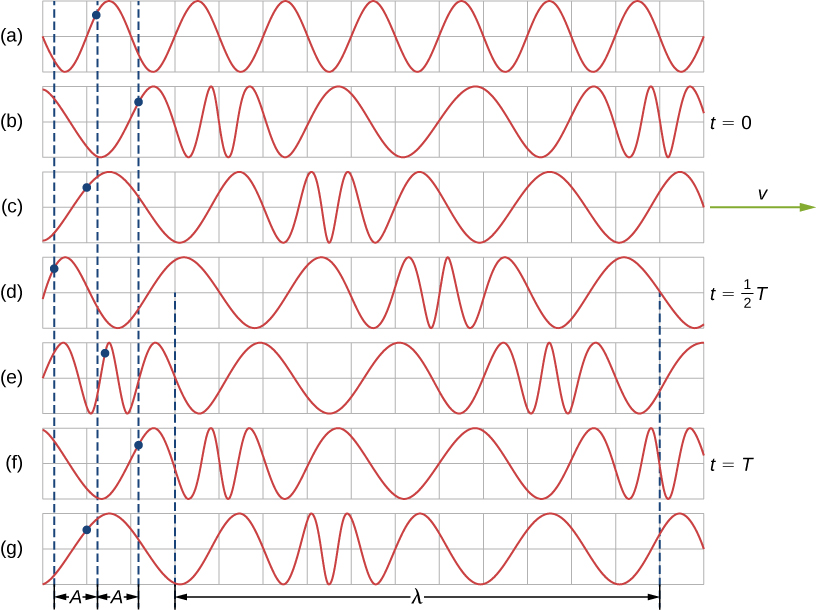
Figure 16.5 (a) This is a simple, graphical representation of a section of the stretched spring shown in (Figure)(b), representing the spring’s equilibrium position before any waves are induced on the spring. A point on the spring is marked by a blue dot. (b–g) Longitudinal waves are created by oscillating the end of the spring (not shown) back and forth along the x-axis. The longitudinal wave, with a wavelength [latex] \lambda [/latex], moves along the spring in the +x-direction with a wave speed v. For convenience, the wavelength is measured in (d). Note that the point on the spring that was marked with the blue dot moves back and forth a distance A from the equilibrium position, oscillating around the equilibrium position of the point.
Waves may be transverse, longitudinal, or a combination of the two. Examples of transverse waves are the waves on stringed instruments or surface waves on water, such as ripples moving on a pond. Sound waves in air and water are longitudinal. With sound waves, the disturbances are periodic variations in pressure that are transmitted in fluids. Fluids do not have appreciable shear strength, and for this reason, the sound waves in them are longitudinal waves. Sound in solids can have both longitudinal and transverse components, such as those in a seismic wave. Earthquakes generate seismic waves under Earth’s surface with both longitudinal and transverse components (called compressional or P-waves and shear or S-waves, respectively). The components of seismic waves have important individual characteristics—they propagate at different speeds, for example. Earthquakes also have surface waves that are similar to surface waves on water. Ocean waves also have both transverse and longitudinal components.
Wave on a String
A student takes a 30.00-m-long string and attaches one end to the wall in the physics lab. The student then holds the free end of the rope, keeping the tension constant in the rope. The student then begins to send waves down the string by moving the end of the string up and down with a frequency of 2.00 Hz. The maximum displacement of the end of the string is 20.00 cm. The first wave hits the lab wall 6.00 s after it was created. (a) What is the speed of the wave? (b) What is the period of the wave? (c) What is the wavelength of the wave?
- The speed of the wave can be derived by dividing the distance traveled by the time.
- The period of the wave is the inverse of the frequency of the driving force.
- The wavelength can be found from the speed and the period [latex] v=\lambda \text{/}T. [/latex]
- The first wave traveled 30.00 m in 6.00 s: [latex] v=\frac{30.00\,\text{m}}{6.00\,\text{s}}=5.00\frac{\text{m}}{\text{s}}. [/latex]
- The period is equal to the inverse of the frequency: [latex] T=\frac{1}{f}=\frac{1}{2.00\,{\text{s}}^{-1}}=0.50\,\text{s}. [/latex]
- The wavelength is equal to the velocity times the period: [latex] \lambda =vT=5.00\frac{\text{m}}{\text{s}}(0.50\,\text{s})=2.50\,\text{m}. [/latex]
Significance
The frequency of the wave produced by an oscillating driving force is equal to the frequency of the driving force.
Check Your Understanding
When a guitar string is plucked, the guitar string oscillates as a result of waves moving through the string. The vibrations of the string cause the air molecules to oscillate, forming sound waves. The frequency of the sound waves is equal to the frequency of the vibrating string. Is the wavelength of the sound wave always equal to the wavelength of the waves on the string?
The wavelength of the waves depends on the frequency and the velocity of the wave. The frequency of the sound wave is equal to the frequency of the wave on the string. The wavelengths of the sound waves and the waves on the string are equal only if the velocities of the waves are the same, which is not always the case. If the speed of the sound wave is different from the speed of the wave on the string, the wavelengths are different. This velocity of sound waves will be discussed in Sound .
Characteristics of a Wave
A transverse mechanical wave propagates in the positive x -direction through a spring (as shown in (Figure) (a)) with a constant wave speed, and the medium oscillates between [latex] \text{+}A [/latex] and [latex] \text{−}A [/latex] around an equilibrium position. The graph in (Figure) shows the height of the spring ( y ) versus the position ( x ), where the x -axis points in the direction of propagation. The figure shows the height of the spring versus the x -position at [latex] t=0.00\,\text{s} [/latex] as a dotted line and the wave at [latex] t=3.00\,\text{s} [/latex] as a solid line. (a) Determine the wavelength and amplitude of the wave. (b) Find the propagation velocity of the wave. (c) Calculate the period and frequency of the wave.
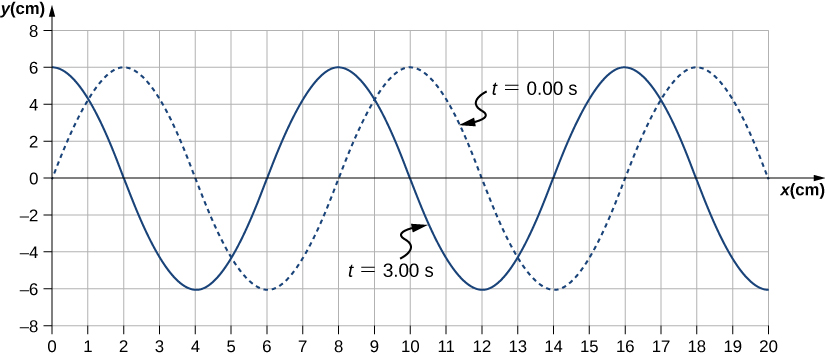
Figure 16.6 A transverse wave shown at two instants of time.
- The amplitude and wavelength can be determined from the graph.
- Since the velocity is constant, the velocity of the wave can be found by dividing the distance traveled by the wave by the time it took the wave to travel the distance.
- The period can be found from [latex] v=\frac{\lambda }{T} [/latex] and the frequency from [latex] f=\frac{1}{T}. [/latex]
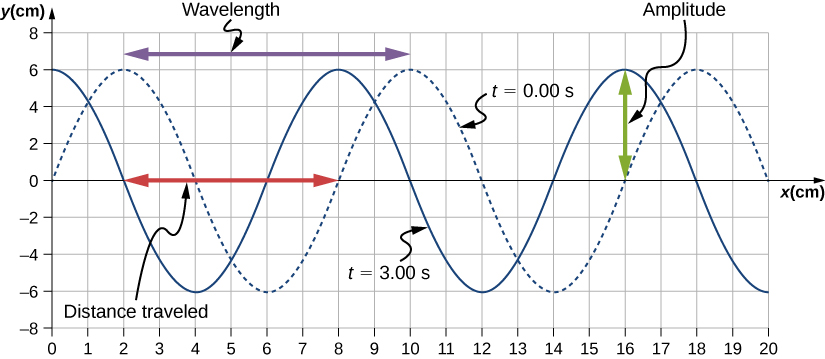
Figure 16.7 Characteristics of the wave marked on a graph of its displacement.
- The distance the wave traveled from time [latex] t=0.00\,\text{s} [/latex] to time [latex] t=3.00\,\text{s} [/latex] can be seen in the graph. Consider the red arrow, which shows the distance the crest has moved in 3 s. The distance is [latex] 8.00\,\text{cm}-2.00\,\text{cm}=6.00\,\text{cm}. [/latex] The velocity is [latex] v=\frac{\text{Δ}x}{\text{Δ}t}=\frac{8.00\,\text{cm}-2.00\,\text{cm}}{3.00\,\text{s}-0.00\,\text{s}}=2.00\,\text{cm/s}. [/latex]
- The period is [latex] T=\frac{\lambda }{v}=\frac{8.00\,\text{cm}}{2.00\,\text{cm/s}}=4.00\,\text{s} [/latex] and the frequency is [latex] f=\frac{1}{T}=\frac{1}{4.00\,\text{s}}=0.25\,\text{Hz}. [/latex]
Note that the wavelength can be found using any two successive identical points that repeat, having the same height and slope. You should choose two points that are most convenient. The displacement can also be found using any convenient point.
The propagation velocity of a transverse or longitudinal mechanical wave may be constant as the wave disturbance moves through the medium. Consider a transverse mechanical wave: Is the velocity of the medium also constant?
- A wave is a disturbance that moves from the point of origin with a wave velocity v .
- A wave has a wavelength [latex] \lambda [/latex], which is the distance between adjacent identical parts of the wave. Wave velocity and wavelength are related to the wave’s frequency and period by [latex] v=\frac{\lambda }{T}=\lambda f. [/latex]
- Mechanical waves are disturbances that move through a medium and are governed by Newton’s laws.
- Electromagnetic waves are disturbances in the electric and magnetic fields, and do not require a medium.
- Matter waves are a central part of quantum mechanics and are associated with protons, electrons, neutrons, and other fundamental particles found in nature.
- A transverse wave has a disturbance perpendicular to the wave’s direction of propagation, whereas a longitudinal wave has a disturbance parallel to its direction of propagation.
Conceptual Questions
Give one example of a transverse wave and one example of a longitudinal wave, being careful to note the relative directions of the disturbance and wave propagation in each.
A sinusoidal transverse wave has a wavelength of 2.80 m. It takes 0.10 s for a portion of the string at a position x to move from a maximum position of [latex] y=0.03\,\text{m} [/latex] to the equilibrium position [latex] y=0. [/latex] What are the period, frequency, and wave speed of the wave?
What is the difference between propagation speed and the frequency of a mechanical wave? Does one or both affect wavelength? If so, how?
Propagation speed is the speed of the wave propagating through the medium. If the wave speed is constant, the speed can be found by [latex] v=\frac{\lambda }{T}=\lambda f. [/latex] The frequency is the number of wave that pass a point per unit time. The wavelength is directly proportional to the wave speed and inversely proportional to the frequency.
Consider a stretched spring, such as a slinky. The stretched spring can support longitudinal waves and transverse waves. How can you produce transverse waves on the spring? How can you produce longitudinal waves on the spring?
Consider a wave produced on a stretched spring by holding one end and shaking it up and down. Does the wavelength depend on the distance you move your hand up and down?
No, the distance you move your hand up and down will determine the amplitude of the wave. The wavelength will depend on the frequency you move your hand up and down, and the speed of the wave through the spring.
A sinusoidal, transverse wave is produced on a stretched spring, having a period T . Each section of the spring moves perpendicular to the direction of propagation of the wave, in simple harmonic motion with an amplitude A . Does each section oscillate with the same period as the wave or a different period? If the amplitude of the transverse wave were doubled but the period stays the same, would your answer be the same?
An electromagnetic wave, such as light, does not require a medium. Can you think of an example that would support this claim?
Storms in the South Pacific can create waves that travel all the way to the California coast, 12,000 km away. How long does it take them to travel this distance if they travel at 15.0 m/s?
Waves on a swimming pool propagate at 0.75 m/s. You splash the water at one end of the pool and observe the wave go to the opposite end, reflect, and return in 30.00 s. How far away is the other end of the pool?
[latex] 2d=vt⇒d=11.25\,\text{m} [/latex]
Wind gusts create ripples on the ocean that have a wavelength of 5.00 cm and propagate at 2.00 m/s. What is their frequency?
How many times a minute does a boat bob up and down on ocean waves that have a wavelength of 40.0 m and a propagation speed of 5.00 m/s?
Scouts at a camp shake the rope bridge they have just crossed and observe the wave crests to be 8.00 m apart. If they shake the bridge twice per second, what is the propagation speed of the waves?
What is the wavelength of the waves you create in a swimming pool if you splash your hand at a rate of 2.00 Hz and the waves propagate at a wave speed of 0.800 m/s?
[latex] v=f\lambda ⇒\lambda =0.400\,\text{m} [/latex]
What is the wavelength of an earthquake that shakes you with a frequency of 10.0 Hz and gets to another city 84.0 km away in 12.0 s?
Radio waves transmitted through empty space at the speed of light [latex] (v=c=3.00\,×\,{10}^{8}\,\text{m/s}) [/latex] by the Voyager spacecraft have a wavelength of 0.120 m. What is their frequency?
Your ear is capable of differentiating sounds that arrive at each ear just 0.34 ms apart, which is useful in determining where low frequency sound is originating from. (a) Suppose a low-frequency sound source is placed to the right of a person, whose ears are approximately 18 cm apart, and the speed of sound generated is 340 m/s. How long is the interval between when the sound arrives at the right ear and the sound arrives at the left ear? (b) Assume the same person was scuba diving and a low-frequency sound source was to the right of the scuba diver. How long is the interval between when the sound arrives at the right ear and the sound arrives at the left ear, if the speed of sound in water is 1500 m/s? (c) What is significant about the time interval of the two situations?
(a) Seismographs measure the arrival times of earthquakes with a precision of 0.100 s. To get the distance to the epicenter of the quake, geologists compare the arrival times of S- and P-waves, which travel at different speeds. If S- and P-waves travel at 4.00 and 7.20 km/s, respectively, in the region considered, how precisely can the distance to the source of the earthquake be determined? (b) Seismic waves from underground detonations of nuclear bombs can be used to locate the test site and detect violations of test bans. Discuss whether your answer to (a) implies a serious limit to such detection. (Note also that the uncertainty is greater if there is an uncertainty in the propagation speeds of the S- and P-waves.)
a. The P-waves outrun the S-waves by a speed of [latex] v=3.20\,\text{km/s;} [/latex] therefore, [latex] \text{Δ}d=0.320\,\text{km}. [/latex] b. Since the uncertainty in the distance is less than a kilometer, our answer to part (a) does not seem to limit the detection of nuclear bomb detonations. However, if the velocities are uncertain, then the uncertainty in the distance would increase and could then make it difficult to identify the source of the seismic waves.
A Girl Scout is taking a 10.00-km hike to earn a merit badge. While on the hike, she sees a cliff some distance away. She wishes to estimate the time required to walk to the cliff. She knows that the speed of sound is approximately 343 meters per second. She yells and finds that the echo returns after approximately 2.00 seconds. If she can hike 1.00 km in 10 minutes, how long would it take her to reach the cliff?
A quality assurance engineer at a frying pan company is asked to qualify a new line of nonstick-coated frying pans. The coating needs to be 1.00 mm thick. One method to test the thickness is for the engineer to pick a percentage of the pans manufactured, strip off the coating, and measure the thickness using a micrometer. This method is a destructive testing method. Instead, the engineer decides that every frying pan will be tested using a nondestructive method. An ultrasonic transducer is used that produces sound waves with a frequency of [latex] f=25\,\text{kHz}. [/latex] The sound waves are sent through the coating and are reflected by the interface between the coating and the metal pan, and the time is recorded. The wavelength of the ultrasonic waves in the coating is 0.076 m. What should be the time recorded if the coating is the correct thickness (1.00 mm)?
- OpenStax University Physics. Authored by : OpenStax CNX. Located at : https://cnx.org/contents/[email protected]:Gofkr9Oy@15 . License : CC BY: Attribution . License Terms : Download for free at http://cnx.org/contents/[email protected]
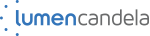
Privacy Policy
The Nature of Waves
A wave is a disturbance that propagates through a medium. There are three words in that definition that may need unpacking: disturbance, propagate, and medium.
- a change in a kinematic variable like position, velocity, or acceleration;
- a change in an intensive property like pressure, density, or temperature;
- a change in field strength like electric field strength, magnetic field strength, or gravitational field strength.
- To propagate , in the sense used in this definition, is to transmit the influence of something in a particular direction. Synonyms for propagate include spread, transmit, communicate, and broadcast. The noun form of the word is propagation .
- A medium is the substance through which a wave can propagate. Water is the medium of ocean waves. Air is the medium through which we hear sound waves. The electric and magnetic fields are the medium of light. People are the medium of a stadium wave. The Earth is the medium of seismic waves (earthquake waves). Cell membranes are the medium of nerve impulses. Transmission lines are the medium of alternating current electric power. Medium is the singuar form of the noun. Media is the plural form (although mediums is prefered by some people).
Let's list a few key examples of wave phenomena and then connect them to this definition. The first example that comes to mind when most people hear the word wave are the kinds of waves that one sees on the surface of a body of water: deep water waves in the ocean or ripples in a puddle. The most important kinds of waves for humans are the waves we use to sense the world around us: sound and light.
Imagine a calm pool. The surface is flat and smooth. Drop a rock into it. Kerploop. The surface is now disturbed. It is higher than normal in some places and lower than normal in others. The disturbed water at the point of impact disturbs the water next to it, which in turn disturbs the water next to it, which disturbs the water next to it, and so on. The disturbance spreads outward, transmits, or propagates. The medium through which this disturbance propagates is the surface of the water.
Imagine a quiet room. The air inside is still. Drop a book onto a table in that room. Thwap. The air between the book and the table is squeezed out in a fraction of a second. The air pressure in that rapidly decreasing gap rises above normal and then rebounds. The rise and fall of pressure is like the rise and fall of the surface of the pool in the previous example. The air under the book bumps the air on the edges of the book, which bumps the air next to it, which bumps the air next to it, and so on. The medium through which this disturbance propagates is the air.
Those were the easy examples. Water waves and sound waves are examples of mechanical waves — waves that propagate through a material medium. Light is not so easy to understand as a wave, which is why there are multiple sections of this book devoted to it. Still, I am going to try to describe it briefly.
Imagine a dark cavern, deep within the Earth. The electric and magnetic fields inside are relatively static and unchanging. Strike a match. Skeerach. The atoms of carbon in the wood of the matchstick combine with the atoms of oxygen in the air releasing heat. The heat agitates the atoms of the combustion products resulting in the phenomenon known as fire. The electrons bound to the rapidly vibrating atoms disturb the electric and magnetic fields in the space surrounding them. These fields are "elastic" in a sense. A wiggle in the fields in one place causes a wiggle in the fields nearby, which causes a wiggle in the fields nearby, and so on. These wiggles eventually make it to your eye, which you perceive as light. The electric and magnetic fields that fill all of space are the medium.
essential property
Waves transfer energy, momentum, and information, but not mass.
A naive description of a wave is that it has something to do with motion. But the motion of a wave on the water is not the same as the motion of the water from a hose. When waves move over the surface of the ocean, where does the ocean go? Nowhere. When waves reach the shore, does the water accumulate into great heaps? No. The water moves in and out, and the ocean stays behind. Even when huge tsunamis strike, the wall of water deposited on the land eventually drains back into the sea. In this case, no net transfer of mass has occurred.
Compare this to the water from a hose. The water comes pouring out the open end and stays where it lands forming a puddle or drains away to some other location. It most certainly does not jump back into the hose. In this case, mass has been transferred from one location to another.
Any sensible person who owns waterfront property should be familiar with the word erosion. Ocean waves (or waves on the Great Lakes for that matter) break on the shore, beating the rock and soil into submission and pulling it away. This material will never return. (If there were no plate tectonic forces lifting the land up in some places or volcanoes creating new land in others, the Earth would be covered in a global sea of uniform depth.) A force ( F ) has been exerted and mass has been displaced ( ∆ s ). Work has been done ( W = F ∆ s ). The ability to do work is one definition of energy ( W = ∆ E ). Thus waves transfer energy.
Sticking with the example of ocean waves, anyone who surfs knows that waves transfer momentum. I have less to say on this subject.
Sound and light are the two primary examples of the way we gather information around us as humans. We have specialized sensory organs called ears and eyes for doing just that.
Here's a list of some phenomena or activities that satisfy the definition of a wave given above.
- including hock waves
- Radio waves
- Visible light
- Ultraviolet
- Tsunamis (tidal waves)
- Ripples (capillary waves)
- P waves (primary waves, pressure waves)
- S waves (secondary waves, shear waves)
- R waves (Rayleigh waves, ground roll)
- L waves (Love waves)
- A fluttering flag
- Snapping a sheet when making a bed
- Nerve impulses
- Peristalsis
- Heart contractions
- Snakes, eels, etc
- Worms, slugs, etc.
- Centipedes, millipedes, caterpillars, etc.
- Plucking, bowing, or striking a guitar, violin, or piano string
- Casting loops when fly fishing
- Cracking a whip
- Gravitational waves (as described in general relativity, not to be confused with gravity waves in water)
- Matter waves (quantum mechanical waves, de Broglie waves)
- Dominoes (as a show, not as a game)
- Stadium wave (Mexican wave, the wave)
- Newton's cradle (paid link)

not examples
Just because the word wave is used doesn't mean the thing being described is a wave in the sense used in this book.
- Waving as a signal to get someone's attention or to greet them or to say goodbye is not a wave. It does not propagate in a direction. Just because you wave at me does not mean that I have to start waving followed by a person behind me and the person behind them and the person behind them.
- A permanent wave set in a person's hair is not a wave. The term is almost an oxymoron. Nothing's moving if a thing is permanent. Also, you getting a wave set in your hair does not result in the people nearest you getting one followed by the people next to them getting one, and so on, until the whole globe is filled with wavy haired people.
- Wheat, or any other tall grass, is sometimes said to wave when gusts of wind pass over it. That bulk flow of matter is not a wave and neither is the response of the wheat.
- A heat wave is a meteorological term referring to a prolonged period of unusually high temperature. This definition has no connection to a phenomenon that propagates. Just because it's hot for a long period in one location does not imply that the heat wave will propagate to another location. It's actually sort of the opposite. A heat wave is often a region where the hot air is "stuck". The opposite of a heat wave could be called a cold wave, but it's usually described a cold snap (at least in the dialect of English I'm used to hearing). This should indicate that neither one of these phenomena is really a wave. Sometimes infrared radiation is described colloquially as heat waves, but that's not the right term. The same goes for rising thermals in the desert. That shimmering effect sometimes seen on the horizon is turbulent air of different density streaming upward and not a wave. Waves do not transfer mass.
- A crime wave is like a heat wave, but for crime. Since heat waves aren't waves, neither are crime waves.
- Making waves, meaning to stir up trouble, is not an example of a wave — and don't you disagree with me you trouble maker.
Classification of waves
Waves can be classified according to the medium through which they propagate.
by the type of disturbance
Waves can be classified according to the type of disturbance — meaning its relative direction or shap. There is a lot that can be said about this organizational scheme. I'm starting this part of this section with a quick summary in table form followed by a rather detailed follow up.
transverse waves
A transverse wave is one in which the direction of the disturbance is perpendicular to the direction of propagation. The word transverse describes something pointing in a sideways or lateral direction. As dynamic phenomena, waves are better represented with animations than with static images. Click on the static image below to see a transverse wave in action.
A cartoon representation of this kind of wave is your classic wiggly line. People with a bit of math knowledge will tell you they drew a sine curve. Those with a little bit more math knowledge will say they drew a sine or cosine curve.
The high parts on a curve like this are called crests . The low parts are called troughs . Since directions like up and down don't always make sense for waves, what the parts really represent are the maximal changes. The points labeled crests are points corresponding to a maximal increase of the changing quantity in a whatever direction is decided to be positive. The points labeled troughs are the points corresponding to the maximal change in the opposite direction.
Pronouncing words ending in -ough in English is often a mystery. The word trough rhymes with off. A trough is what one uses to provide food and water to livestock and other domesticated animals — typically a long, narrow open container that an animal would dip its head down into. The word crest rhymes with best. A crest is something at the top of something. Many birds, usually male birds, have crests. Hills and mountains are are sometimes said to have crests. The crest on a men's sports jacket or a school uniform gets its name from the heraldic crests that were originally worn on knight's helmets above the visor. Crests are up high. Troughs are down low.
The most important example of a transverse wave for humans is light. Most of what I am about to say in the following bullets will really be discussed elsewhere in this book.
- Showing that light is a just wave was not easy before the 20th century. Now that we have easy access to lasers in the 21st century, it's less of a problem. Light can be made to interfere with itself and produce a pattern of what are called interference fringes . A laser and two or more closely spaced openings are all that is needed. The iridescence seen when gasoline is spilled on water, in some insects like scarab beetles and butterflies, and in pearls and the shells of mollusks is caused by thin film interference . Observing this wave behavior of light requires no special technology.
- Showing that light is a transverse wave was was also not easy before the 20th century. Now that we have easy access to polarized sunglasses and polarized electronic displays in the 21st century, it's less of a problem. Light can be shown to exhibit polarization . Try looking at certain electronic displays with polarized sunglasses. If the orientation of the sunglasses is perpendicular to the orientation of the display, the display will look dark (or really screwed up). If the orientation is parallel, the display will look normal (or closer to normal than when they were perpendicular).
Lots of musical instruments make use of transverse waves to generate their characteristic sound.
- The source of the sound that comes out of violins, guitars, pianos and other chordophones is the side to side motion of a nylon or metal string (or in the olden days, dried animal intestines). The parts of the string vibrate side to side, but the wave travels along the length of the string. These two directions are perpendicular, which makes the waves transverse. The vibrations of the string are also transmitted into the wooded bodies or sound boards of these instruments. These flat wooden parts are driven to flex in and out, but the energy propagates across the surface. The two directions here are also perpendicular.
- The source of the sound that comes out of drum heads, kazoos or other membranophones is the in and out vibration of some flat, membrane-like structure. The waves produced by striking, stroking, or humming into these devices generates waves that crisscross the object. Once again, the disturbance is perpendicular to the propagation.
- Most percussion instruments that are not drums are classified as idiophones . Cymbals, triangles, and xylophones produce sound by the vibration of the entire object or a piece that is not a string, membrane, or column of air. The waves set up in many of these instruments are transverse, but because the class is so large and varied there is probably an exception out there somewhere.
Some animals propel themselves by sending transverse waves down the length of their bodies.
- Fish use a variety of means for getting around but long, thin, tubular fish like eels, lamprey, and dogfish are what comes to mind when I think about locomotion by transverse waves. A wave of side to side motion starts at the head and propagates backward along the spine. This propels the fish forward.
- Snakes also have several mechanisms for propelling themselves. The one that is considered most "normal" is called lateral undulation and has the classic look of a transverse wave in a one-dimensional medium. Much like the fish described above, a wave starts at the head as side to side motion and propagates backward down the length of the snake. A fancier kind of locomotion that relies on transverse waves is called sidewinding and the snakes that use it live in sandy deserts or slippery mud flats — anywhere getting a good grip on the ground is difficult. It's still an example of a transverse wave, but it propels the snake diagonally instead of forward relative to its body axis.
longitudinal waves
- pressure, compression, density
- aerophones - vibrating columns of air (horns, whistles, organ pipes )
- primary (P) pressure
- invertebrates (worms)
- traffic jams
- density waves in spiral galaxies generate the arms
- compressions (a.k.a. condensation): the pressed part, the greatest positive pressure change, a region where the medium is under compression
- rarefactions (a.k.a. dilations): the stretched part, the lowest negative pressure change, a region where the medium is under tension
complex waves
classed by orientation of change
- P rimary (surface, compression, P ressure)
- S econdary (transverse, S hear), can't propagate through liquids
- L ove, ( L ateral shear)
- R ayleigh, (elliptical, plate waves, ground R oll), something like ocean waves, but elliptical instead of circular
torsional waves
By duration.
classified by duration
by appearance
Waves can be classified according to what they appear to be doing.
Now look at these pretty, moving pictures.
EN - English
DE - Deutsch
ES - Español
IT - Italiano
FR - Français
PT - Português
Table of content
Full table of contents
A wave is a disturbance that propagates from its source, repeating itself periodically, and is typically associated with simple harmonic motion. Mechanical waves are governed by Newton's laws and require a medium to travel. A medium is a substance in which a mechanical wave propagates, and the medium produces an elastic restoring force when it is deformed.
Water waves, sound waves, and seismic waves are some examples of mechanical waves. For water waves, the wave propagation medium is water; for sound waves, the medium is usually air, but could also be water. The wave disturbance in sound waves is generally a change in air pressure. In the case of mechanical waves, both energy and momentum are transferred with the wave's motion, whereas the mass oscillates around an equilibrium point.
Waves can be transverse, longitudinal, or a combination of the two. For example, the waves on the strings of musical instruments are transverse. Meanwhile, sound waves in air and water are longitudinal; their disturbances are periodic variations in pressure that are transmitted in fluids. Fluids do not have appreciable shear strength, and thus, the sound waves in them must be longitudinal or compressional. However, sound waves that travel in solids can be both longitudinal and transverse.
Earthquake waves under the Earth's surface also have both longitudinal and transverse components. These components have important individual characteristics—they propagate at different speeds. Earthquakes also have surface waves that are similar to surface waves on water.
This text is adapted from Openstax, University Physics Volume 1, Section 16.1: Traveling Waves.
Terms of Use
Recommend to library
JoVE NEWSLETTERS
JoVE Journal
Methods Collections
JoVE Encyclopedia of Experiments
JoVE Science Education
JoVE Lab Manual
Faculty Resource Center
Publishing Process
Editorial Board
Scope and Policies
Peer Review
Testimonials
Subscriptions
Library Advisory Board
Copyright © 2024 MyJoVE Corporation. All rights reserved
Talk to our experts
1800-120-456-456
- Travelling Wave
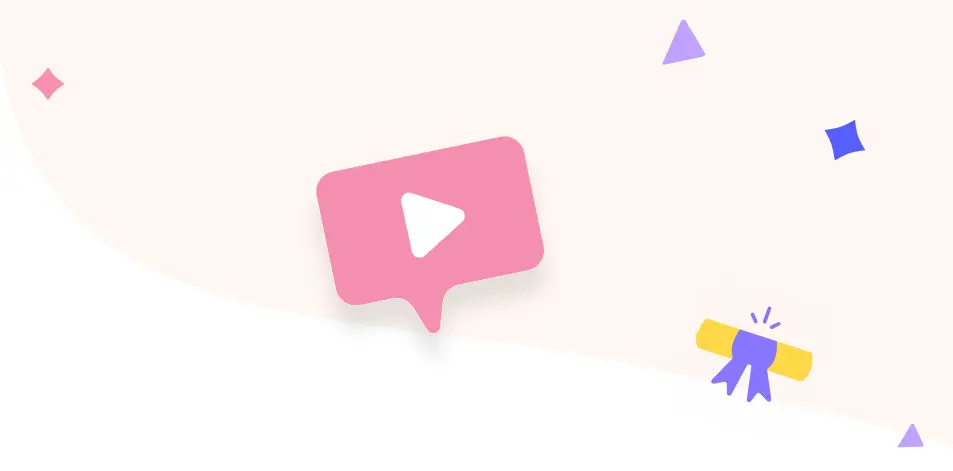
Have you ever sat by a lake and observed the waves created on the surface of the water when you throw a stone into it? This is a good visual example of the propagation of waves and makes it simpler for you to understand travelling of waves and all other concepts related to it. Our universe has an amazing way of informing us about any changes in the physical world. When there are changes the information about that disturbance moves gradually outwards. It moves far from the source of disturbance in all the directions. When the said information travels, it travels in the form of a wave, just like the way waves are created when you throw a stone in the still water. This is known as the travelling wave.
Define Travelling Wave
(image will be uploaded soon)
Before understanding what is travelling wave, let’s understand waves. Wave can be defined as a disturbance in a medium that travels transferring momentum and energy without any actual movement of the medium. However, the medium must have elastic properties. In our everyday life, there are many examples of waves, for example, ocean waves, strings of musical instruments, etc. On the other hand, a travelling wave is a wave in which the positions of minimum and maximum amplitude travel through the medium.
Points To Remember
Here are some of the points that are necessary to keep in mind about the wave:
Every wave has a high point and a low point. The high points are known by the name of crests. On the other hand, the low points are named by troughs.
Amplitude is the maximum distance of the disturbance from the midpoint of the wave to either the top of the crest or the bottom of a trough.
The maximum distance between the two adjacent troughs or the two adjacent crests is known as a wavelength.
Now, the time period is actually the time taken to complete one vibration.
Frequency is the number of vibrations the wave undergoes in one second.
You can witness an inverse relationship between both frequency and time period. The relationship is given below,
The speed of a wave is given by the travelling wave equation,
Where 𝛌 is the wavelength.
What are the Various Types of Travelling Waves?
Each type of wave contains different characteristics. And with these characteristics, we can easily distinguish between them. Here is a list of different types of waves that have been categorized based upon their particle motion.
Pulse Waves - the sudden disturbance that travels through a medium is known as a pulse wave. The disturbance can be caused by a chain reaction or sudden compression of air caused by an explosion. One example of a pulse wave is thunder. It comprises only one crest that travels through the transmission medium.
Continuous Waves - it is an electromagnetic wave that has constant amplitude and frequency. It is a typical sine wave and is considered to be of infinite duration. It was used in the earlier days of radio transmission.
Transverse Waves - in the transverse wave, the movement of the particles is at right angles to the motion of the energy. It is generated through a solid object like a stretched rope. Trampoline is the best example to understand this wave.
Longitudinal Waves - in this type of travelling wave the motion of the wave-particle is in the same direction as the propagation of the wave. In simple words, the movement of the particles is parallel to the motion of the energy. The best example for longitudinal waves is sound waves moving through the air when you hear a loudspeaker playing in the distance.
There is a second way to characterize the waves by types of matter they are able to move or travel through.
Electromagnetic Waves - this type of wave can travel easily through a vacuum. It does not need any medium, soft or hard to travel. An example of an electromagnetic wave is mobile phone waves or sound waves. They don't need any vacuum to travel.
Physical waves - Unlike electromagnetic waves, they require a medium to travel. They are further distinguished on the basis of phases of matter through which they can move.
Longitudinal Waves - these waves can easily pass through liquids and games.
Transverse Waves - they require a solid material or medium to propagate.
Problems based on travelling wave equation
Solved Examples
1: A wave on a rope is shown on the right at some time t. What is the wavelength of this wave? If the said frequency is about 4 Hz, what will be the wave speed?
Now, for all the periodic waves, you will find v = λ/T = λf.
Details of the calculation:
The wavelength λ is 3 m. The speed is v = λf = (3 m)(4/s) = 12 m/s.
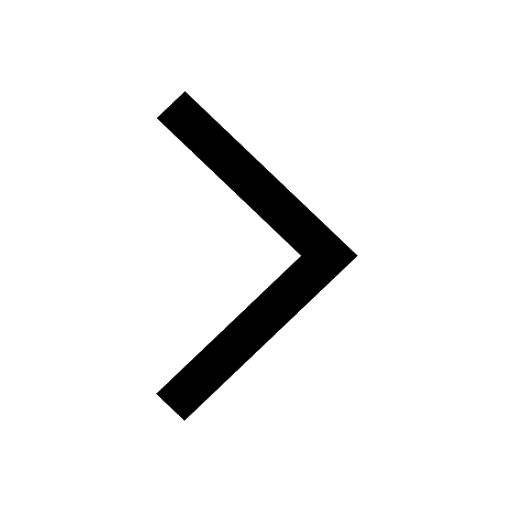
FAQs on Travelling Wave
1. Do all the Waves have a Defined Wavelength?
Yes, every wave has a specific wavelength, which is defined as the length from one wave crest to the next.
Different kinds of waves have different wavelengths. In water, the surf waves produced by a surfer, have wavelengths of 30–50 m, and the large tsunamis have much longer wavelengths (about 100km). The Sound waves vary in wavelength according to the pitch of it.
2. At What Wavelengths can a Human Hear a Sound?
As per experts and facts, the humans can very well hear sounds possessing wavelengths between 70 mm and 70 m. Any sound above or below this level can not be heard by a human. Example- our universe is filled with cosmic noises. The wavelength of the noise is so high that we cannot hear it on earth.
16.1: Travelling Waves
Chapter 1: units, dimensions, and measurements, chapter 2: vectors and scalars, chapter 3: motion along a straight line, chapter 4: motion in two or three dimensions, chapter 5: newton's laws of motion, chapter 6: application of newton's laws of motion, chapter 7: work and kinetic energy, chapter 8: potential energy and energy conservation, chapter 9: linear momentum, impulse and collisions, chapter 10: rotation and rigid bodies, chapter 11: dynamics of rotational motions, chapter 12: equilibrium and elasticity, chapter 13: fluid mechanics, chapter 14: gravitation, chapter 15: oscillations, chapter 16: waves, chapter 17: sound, chapter 18: temperature and heat, chapter 19: the kinetic theory of gases, chapter 20: the first law of thermodynamics, chapter 21: the second law of thermodynamics, chapter 22: electric charges and fields, chapter 23: gauss's law, chapter 24: electric potential, chapter 25: capacitance, chapter 26: current and resistance, chapter 27: direct-current circuits, chapter 28: magnetic forces and fields, chapter 29: sources of magnetic fields, chapter 30: electromagnetic induction, chapter 31: inductance, chapter 32: alternating-current circuits, chapter 33: electromagnetic waves.
The JoVE video player is compatible with HTML5 and Adobe Flash. Older browsers that do not support HTML5 and the H.264 video codec will still use a Flash-based video player. We recommend downloading the newest version of Flash here, but we support all versions 10 and above.

When a stone is thrown on the surface of a calm lake, we see circular rings form and spread out from the drop point. These circular rings observed on the water surface are an example of wave motion.
Wave motion is a disturbance that propagates from a state of rest or equilibrium without bulk motion of matter.
Traveling waves can be divided into transverse waves and longitudinal waves.
In a longitudinal wave, the particles of the propagating medium displace parallel to the wave propagation in back and forth motion.
Sound waves traveling in air or the sound waves traveling in water are examples of longitudinal waves.
Whereas, in a transverse wave, the particle displacement is perpendicular to the direction of wave propagation.
Ripples on the surface of the water and the waves produced from the stretched string of a guitar are examples of transverse waves.
A wave is a disturbance that propagates from its source, repeating itself periodically, and is typically associated with simple harmonic motion. Mechanical waves are governed by Newton's laws and require a medium to travel. A medium is a substance in which a mechanical wave propagates, and the medium produces an elastic restoring force when it is deformed.
Water waves, sound waves, and seismic waves are some examples of mechanical waves. For water waves, the wave propagation medium is water; for sound waves, the medium is usually air, but could also be water. The wave disturbance in sound waves is generally a change in air pressure. In the case of mechanical waves, both energy and momentum are transferred with the wave's motion, whereas the mass oscillates around an equilibrium point.
Waves can be transverse, longitudinal, or a combination of the two. For example, the waves on the strings of musical instruments are transverse. Meanwhile, sound waves in air and water are longitudinal; their disturbances are periodic variations in pressure that are transmitted in fluids. Fluids do not have appreciable shear strength, and thus, the sound waves in them must be longitudinal or compressional. However, sound waves that travel in solids can be both longitudinal and transverse.
Earthquake waves under the Earth's surface also have both longitudinal and transverse components. These components have important individual characteristics—they propagate at different speeds. Earthquakes also have surface waves that are similar to surface waves on water.
This text is adapted from Openstax, University Physics Volume 1, Section 16.1: Traveling Waves.
Get cutting-edge science videos from J o VE sent straight to your inbox every month.
mktb-description
We use cookies to enhance your experience on our website.
By continuing to use our website or clicking “Continue”, you are agreeing to accept our cookies.

If you're seeing this message, it means we're having trouble loading external resources on our website.
If you're behind a web filter, please make sure that the domains *.kastatic.org and *.kasandbox.org are unblocked.
To log in and use all the features of Khan Academy, please enable JavaScript in your browser.
Middle school physics - NGSS
Course: middle school physics - ngss > unit 4.
- Wave transmission
- Refraction and frequency
- Refraction in a glass of water
Transmission and refraction
- Understand: transmission and refraction
Key points:
- Transmission happens when a wave travels through a medium or into a new medium .
- Transmission depends on the type of wave, type of material and even the frequency of the wave.
- For example, if you knock on a door the vibration you create will transmit through the door and be heard as sound. However, light does not transmit through a door so you cannot be seen through it.
- Usually, waves travel in a straight line until they interact with a boundary. Refraction happens when a wave’s path bends at a boundary as it transmits into a new medium.
- The amount a wave refracts depends on the type of wave, the frequency of the wave, and the material it is moving into.
Want to join the conversation?
- Upvote Button navigates to signup page
- Downvote Button navigates to signup page
- Flag Button navigates to signup page

13.2 Wave Properties: Speed, Amplitude, Frequency, and Period
Section learning objectives.
By the end of this section, you will be able to do the following:
- Define amplitude, frequency, period, wavelength, and velocity of a wave
- Relate wave frequency, period, wavelength, and velocity
- Solve problems involving wave properties
Teacher Support
The learning objectives in this section will help your students master the following standards:
- (B) investigate and analyze the characteristics of waves, including velocity, frequency, amplitude, and wavelength, and calculate using the relationship between wave speed, frequency, and wavelength;
- (D) investigate the behaviors of waves, including reflection, refraction, diffraction, interference, resonance, and the Doppler effect.
Section Key Terms
[BL] [OL] [AL] Review amplitude, period, and frequency for simple harmonic motion.
Wave Variables
In the chapter on motion in two dimensions, we defined the following variables to describe harmonic motion:
- Amplitude—maximum displacement from the equilibrium position of an object oscillating around such equilibrium position
- Frequency—number of events per unit of time
- Period—time it takes to complete one oscillation
For waves, these variables have the same basic meaning. However, it is helpful to word the definitions in a more specific way that applies directly to waves:
- Amplitude—distance between the resting position and the maximum displacement of the wave
- Frequency—number of waves passing by a specific point per second
- Period—time it takes for one wave cycle to complete
In addition to amplitude, frequency, and period, their wavelength and wave velocity also characterize waves. The wavelength λ λ is the distance between adjacent identical parts of a wave, parallel to the direction of propagation. The wave velocity v w v w is the speed at which the disturbance moves.
Tips For Success
Wave velocity is sometimes also called the propagation velocity or propagation speed because the disturbance propagates from one location to another.
Consider the periodic water wave in Figure 13.7 . Its wavelength is the distance from crest to crest or from trough to trough. The wavelength can also be thought of as the distance a wave has traveled after one complete cycle—or one period. The time for one complete up-and-down motion is the simple water wave’s period T . In the figure, the wave itself moves to the right with a wave velocity v w . Its amplitude X is the distance between the resting position and the maximum displacement—either the crest or the trough—of the wave. It is important to note that this movement of the wave is actually the disturbance moving to the right, not the water itself; otherwise, the bird would move to the right. Instead, the seagull bobs up and down in place as waves pass underneath, traveling a total distance of 2 X in one cycle. However, as mentioned in the text feature on surfing, actual ocean waves are more complex than this simplified example.
Watch Physics
Amplitude, period, frequency, and wavelength of periodic waves.
This video is a continuation of the video “Introduction to Waves” from the "Types of Waves" section. It discusses the properties of a periodic wave: amplitude, period, frequency, wavelength, and wave velocity.
The crest of a wave is sometimes also called the peak .
The Relationship between Wave Frequency, Period, Wavelength, and Velocity
Since wave frequency is the number of waves per second, and the period is essentially the number of seconds per wave, the relationship between frequency and period is
just as in the case of harmonic motion of an object. We can see from this relationship that a higher frequency means a shorter period. Recall that the unit for frequency is hertz (Hz), and that 1 Hz is one cycle—or one wave—per second.
The speed of propagation v w is the distance the wave travels in a given time, which is one wavelength in a time of one period. In equation form, it is written as
From this relationship, we see that in a medium where v w is constant, the higher the frequency, the smaller the wavelength. See Figure 13.8 .
[BL] For sound, a higher frequency corresponds to a higher pitch while a lower frequency corresponds to a lower pitch. Amplitude corresponds to the loudness of the sound.
[BL] [OL] Since sound at all frequencies has the same speed in air, a change in frequency means a change in wavelength.
[Figure Support] The same speaker is capable of reproducing both high- and low-frequency sounds. However, high frequencies have shorter wavelengths and are hence best reproduced by a speaker with a small, hard, and tight cone (tweeter), whereas lower frequencies are best reproduced by a large and soft cone (woofer).
These fundamental relationships hold true for all types of waves. As an example, for water waves, v w is the speed of a surface wave; for sound, v w is the speed of sound; and for visible light, v w is the speed of light. The amplitude X is completely independent of the speed of propagation v w and depends only on the amount of energy in the wave.
Waves in a Bowl
In this lab, you will take measurements to determine how the amplitude and the period of waves are affected by the transfer of energy from a cork dropped into the water. The cork initially has some potential energy when it is held above the water—the greater the height, the higher the potential energy. When it is dropped, such potential energy is converted to kinetic energy as the cork falls. When the cork hits the water, that energy travels through the water in waves.
- Large bowl or basin
- Cork (or ping pong ball)
- Measuring tape
Instructions
- Fill a large bowl or basin with water and wait for the water to settle so there are no ripples.
- Gently drop a cork into the middle of the bowl.
- Estimate the wavelength and the period of oscillation of the water wave that propagates away from the cork. You can estimate the period by counting the number of ripples from the center to the edge of the bowl while your partner times it. This information, combined with the bowl measurement, will give you the wavelength when the correct formula is used.
- Remove the cork from the bowl and wait for the water to settle again.
- Gently drop the cork at a height that is different from the first drop.
- Repeat Steps 3 to 5 to collect a second and third set of data, dropping the cork from different heights and recording the resulting wavelengths and periods.
- Interpret your results.
- No, only the amplitude is affected.
- Yes, the wavelength is affected.
Students can measure the bowl beforehand to help them make a better estimation of the wavelength.
Links To Physics
Geology: physics of seismic waves.
Geologists rely heavily on physics to study earthquakes since earthquakes involve several types of wave disturbances, including disturbance of Earth’s surface and pressure disturbances under the surface. Surface earthquake waves are similar to surface waves on water. The waves under Earth’s surface have both longitudinal and transverse components. The longitudinal waves in an earthquake are called pressure waves (P-waves) and the transverse waves are called shear waves (S-waves). These two types of waves propagate at different speeds, and the speed at which they travel depends on the rigidity of the medium through which they are traveling. During earthquakes, the speed of P-waves in granite is significantly higher than the speed of S-waves. Both components of earthquakes travel more slowly in less rigid materials, such as sediments. P-waves have speeds of 4 to 7 km/s, and S-waves have speeds of 2 to 5 km/s, but both are faster in more rigid materials. The P-wave gets progressively farther ahead of the S-wave as they travel through Earth’s crust. For that reason, the time difference between the P- and S-waves is used to determine the distance to their source, the epicenter of the earthquake.
We know from seismic waves produced by earthquakes that parts of the interior of Earth are liquid. Shear or transverse waves cannot travel through a liquid and are not transmitted through Earth’s core. In contrast, compression or longitudinal waves can pass through a liquid and they do go through the core.
All waves carry energy, and the energy of earthquake waves is easy to observe based on the amount of damage left behind after the ground has stopped moving. Earthquakes can shake whole cities to the ground, performing the work of thousands of wrecking balls. The amount of energy in a wave is related to its amplitude. Large-amplitude earthquakes produce large ground displacements and greater damage. As earthquake waves spread out, their amplitude decreases, so there is less damage the farther they get from the source.
Grasp Check
What is the relationship between the propagation speed, frequency, and wavelength of the S-waves in an earthquake?
- The relationship between the propagation speed, frequency, and wavelength is v w = f λ . v w = f λ .
- The relationship between the propagation speed, frequency, and wavelength is v w = λ f . v w = λ f .
Virtual Physics
Wave on a string.
In this animation, watch how a string vibrates in slow motion by choosing the Slow Motion setting. Select the No End and Manual options, and wiggle the end of the string to make waves yourself. Then switch to the Oscillate setting to generate waves automatically. Adjust the frequency and the amplitude of the oscillations to see what happens. Then experiment with adjusting the damping and the tension.
Which of the settings—amplitude, frequency, damping, or tension—changes the amplitude of the wave as it propagates? What does it do to the amplitude?
- Frequency; it decreases the amplitude of the wave as it propagates.
- Frequency; it increases the amplitude of the wave as it propagates.
- Damping; it decreases the amplitude of the wave as it propagates.
- Damping; it increases the amplitude of the wave as it propagates.
Solving Wave Problems
Worked example, calculate the velocity of wave propagation: gull in the ocean.
Calculate the wave velocity of the ocean wave in the previous figure if the distance between wave crests is 10.0 m and the time for a seagull to bob up and down is 5.00 s.
The values for the wavelength ( λ = 10.0 m ) ( λ = 10.0 m ) and the period ( T = 5.00 s) ( T = 5.00 s) are given and we are asked to find v w v w Therefore, we can use v w = λ T v w = λ T to find the wave velocity.
Enter the known values into v w = λ T v w = λ T
This slow speed seems reasonable for an ocean wave. Note that in the figure, the wave moves to the right at this speed, which is different from the varying speed at which the seagull bobs up and down.
Calculate the Period and the Wave Velocity of a Toy Spring
The woman in Figure 13.3 creates two waves every second by shaking the toy spring up and down. (a)What is the period of each wave? (b) If each wave travels 0.9 meters after one complete wave cycle, what is the velocity of wave propagation?
Strategy FOR (A)
To find the period, we solve for T = 1 f T = 1 f , given the value of the frequency ( f = 2 s − 1 ). ( f = 2 s − 1 ).
Enter the known value into T = 1 f T = 1 f
Strategy FOR (B)
Since one definition of wavelength is the distance a wave has traveled after one complete cycle—or one period—the values for the wavelength ( λ = 0.9 m ) ( λ = 0.9 m ) as well as the frequency are given. Therefore, we can use v w = f λ v w = f λ to find the wave velocity.
Enter the known values into v w = f λ v w = f λ
v w = f λ = ( 2 s −1 )(0 .9 m) = 1 .8 m/s . v w = f λ = ( 2 s −1 )(0 .9 m) = 1 .8 m/s .
We could have also used the equation v w = λ T v w = λ T to solve for the wave velocity since we already know the value of the period ( T = 0.5 s) ( T = 0.5 s) from our calculation in part (a), and we would come up with the same answer.
Practice Problems
The frequency of a wave is 10 Hz. What is its period?
- The period of the wave is 100 s.
- The period of the wave is 10 s.
- The period of the wave is 0.01 s.
- The period of the wave is 0.1 s.
What is the velocity of a wave whose wavelength is 2 m and whose frequency is 5 Hz?
Check Your Understanding
Use these questions to assess students’ achievement of the section’s Learning Objectives. If students are struggling with a specific objective, these questions will help identify such objective and direct them to the relevant content.
What is the amplitude of a wave?
- A quarter of the total height of the wave
- Half of the total height of the wave
- Two times the total height of the wave
- Four times the total height of the wave
- The wavelength is the distance between adjacent identical parts of a wave, parallel to the direction of propagation.
- The wavelength is the distance between adjacent identical parts of a wave, perpendicular to the direction of propagation.
- The wavelength is the distance between a crest and the adjacent trough of a wave, parallel to the direction of propagation.
- The wavelength is the distance between a crest and the adjacent trough of a wave, perpendicular to the direction of propagation.
- f = ( 1 T ) 2
- f = ( T ) 2
When is the wavelength directly proportional to the period of a wave?
- When the velocity of the wave is halved
- When the velocity of the wave is constant
- When the velocity of the wave is doubled
- When the velocity of the wave is tripled
As an Amazon Associate we earn from qualifying purchases.
This book may not be used in the training of large language models or otherwise be ingested into large language models or generative AI offerings without OpenStax's permission.
Want to cite, share, or modify this book? This book uses the Creative Commons Attribution License and you must attribute Texas Education Agency (TEA). The original material is available at: https://www.texasgateway.org/book/tea-physics . Changes were made to the original material, including updates to art, structure, and other content updates.
Access for free at https://openstax.org/books/physics/pages/1-introduction
- Authors: Paul Peter Urone, Roger Hinrichs
- Publisher/website: OpenStax
- Book title: Physics
- Publication date: Mar 26, 2020
- Location: Houston, Texas
- Book URL: https://openstax.org/books/physics/pages/1-introduction
- Section URL: https://openstax.org/books/physics/pages/13-2-wave-properties-speed-amplitude-frequency-and-period
© Jan 19, 2024 Texas Education Agency (TEA). The OpenStax name, OpenStax logo, OpenStax book covers, OpenStax CNX name, and OpenStax CNX logo are not subject to the Creative Commons license and may not be reproduced without the prior and express written consent of Rice University.
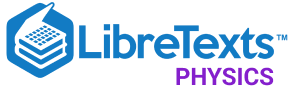
- school Campus Bookshelves
- menu_book Bookshelves
- perm_media Learning Objects
- login Login
- how_to_reg Request Instructor Account
- hub Instructor Commons
- Download Page (PDF)
- Download Full Book (PDF)
- Periodic Table
- Physics Constants
- Scientific Calculator
- Reference & Cite
- Tools expand_more
- Readability
selected template will load here
This action is not available.
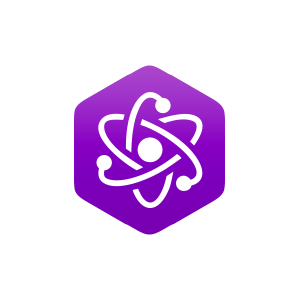
8.1: Introduction to Waves
- Last updated
- Save as PDF
- Page ID 63867
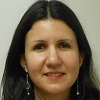
- Dina Zhabinskaya
We begin our study of waves in this first unit of Physics 7C with an introduction to waves and then a thorough development of the harmonic plane wave model , which we will use extensively to model and understand a wide variety of wave phenomena.
In this section we will familiarize ourselves with waves by focusing on material waves . These are the disturbances of atoms or molecules in a particular substance. Any sort of ripple that you have seen is a material wave. Some examples include ripples in a pond or a wave on a slinky or a string. Although not visible to the naked eye, sound is modeled by material waves as well.
One of the distinguishing features of physics is that physicists continually strive for general principles and simple models that can be applied to large classes of phenomena. In our study of wave phenomena we consciously take this approach. The focus is on the models and their representations, not on any one of an almost unlimited number of individual examples associated with waves (like sound, light, TV and radio waves, microwaves, etc.) Our goal is to enable you to develop a useful understanding of wave behavior that you can apply to any phenomenon that can be modeled as a wave.
Basic Wave Concepts
There are two important goals associated with the first part of this unit. Firstly, to become familiar with wave phenomena and how we analyze them, and secondly, to sufficiently understand the mathematical representation of one-dimensional harmonic waves . We want to use this mathematical representation as a tool throughout the rest of the course to help us understand the physics of sound, light, and other types of waves.
A material wave is a very common type of internal motion of a material substance. That substance through which the wave propagates is called the medium . In order for material waves to exist there must be forces between neighboring particles in the medium. For simplicity will examine how a disturbance travels and gives rise to wave-like behavior by coloring a medium three different pieces and labeling them as sections 1, 2 and 3 in Figure 8.1.1 below.
Figure 8.1.1: Representation of a Material Wave
The medium is in equilibrium when it is not being disturbed. If the medium above represents a segment of a rope, then it would lie flat in equilibrium. One can disturb this medium by shaking one end of the rope. In the instant of the wave depicted in Figure 8.1.1 , section 2 is displaced from equilibrium as it pulls on sections 1 and 3. The distance from equilibrium is known as the displacement of the medium as the waves passes through it. By Newton's third law, section 2 is pulled down by sections 1 and 3. This is shown by the opposite arrows,\(F_{\text{1 on 2}}=-F_{\text{2 on 1}}\) and \(F_{\text{3 on 2}}=-F_{\text{2 on 3}}\), in the force diagrams above. Thus, section 2 will accelerate downward, back toward equilibrium. This will cause section section 3 to accelerate upward, so a little time later section 3 is displaced like section 2 was. In this way, the disturbance has traveled from section 2 to section 3 without the individual pieces of medium traveling along with it.
When we describe waves we are describing some kind of motion. We will often speak of the waves moving in space to the right, to the left, or outward from the source. This motion in space, does not refer to the particles which comprise the medium. It is the disturbance or the energy which propagates, defined as the wave. This disturbance occurs due to the interaction between neighboring particles in the medium. These particles oscillate about equilibrium in a wavelike manner, but do not physically travel in space along the wave. In other words, material waves provide a mechanism for transferring energy over considerable distances, without the transport of the medium itself.
You may wonder why the sections exert a force on one another at all. The origin of this force can be traced back to the pairwise interactions you learned about in 7A. Individual atoms have a preferred equilibrium separation distance, and resist being pushed or pulled to maintain that preferred distance. Stretching or compressing the medium causes the atoms to exert forces on their neighbors and to resist forces exerted on them, known as restoring forces. We have simply clumped atoms together into three sections for convenience, but this same discussion applies to individual atoms. The restoring forces responsible for wave behavior are typically strongest in solid materials and negligible in most gaseous materials.
The concepts we introduce when discussing material waves are no different from the concepts that we have already introduced when discussing forces, motion, and atoms. We place such an emphasis on waves as a separate phenomenon because dealing with the form of a wave is often simpler than trying to visualize force diagrams for many different sections of a medium. Eventually we will encounter non-material waves, and this previous exposure to disturbances in material waves will be an invaluable guide.
The disturbance (or, more technically, an oscillation ) may be spread throughout the medium and recur continuously at each point (called a periodic wave ), or the oscillations may exist for only a limited time at each point (a wave pulse ). For example, if you shake one end of a rope depicted in Figure 8.1.1 only one, you will send a wave pulse down the rope. On the other hand, if you continue to shake the rope in a consistent and periodic manner, then you will generate a periodic wave on the rope.
Waves in a medium are started by outside forces that act on some of the particles in the medium to start them oscillating. The object which generates these external forces is known as the source of the wave. External forces are not required to keep a wave going once it has been started. Consider a water wave on the surface of a pond; a rock dropped into the pond is the outside disturbance that starts wave motion. Once started, the ripples expand outward on their own. We don’t have to continue dropping rocks into the pond to keep the ripples moving.
Formal Definition of Waves
It is difficult to come up with a general definition for a "wave". For the moment we will content ourselves by writing down the following definition of a material wave:
A material wave is the large movement of a disturbance in a medium, whereas the particles that make up the medium oscillate about a fixed equilibrium position .
Let’s try again to understand what exactly is moving when describing a wave through an example. Consider ripples expanding in a pond. While there is some movement of the individual water molecules, they merely bob up and down, and they do not travel. It is the ripples that move significantly. The movement of the ripples across the surface of the water is what we mean by a wave. It is not the movement of the individual water molecules, or the disturbance of the surface of water from equilibrium, but rather the movement of the disturbance to different locations in the medium that we call the wave.
Try an experiment at home to clarify this distinction of motion when describing a wave. Take a bowl of water, and drop a small amount of olive oil on the surface, so that you have a set-up like picture below. If you oscillate your hand gently at the location \(x\), do you get ripples at the location \(y\)? What about the oil? Does it move to the location \(y\)?
Figure 8.1.2: Wave Motion Experiment
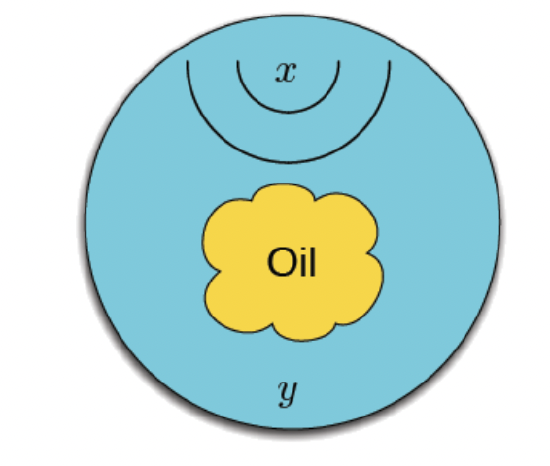
A pulse is a disturbance with a finite length. For example, if you shook the end of a rope only once you would produce a pulse wave as shown below.
Figure 8.1.3: Wave Pulse
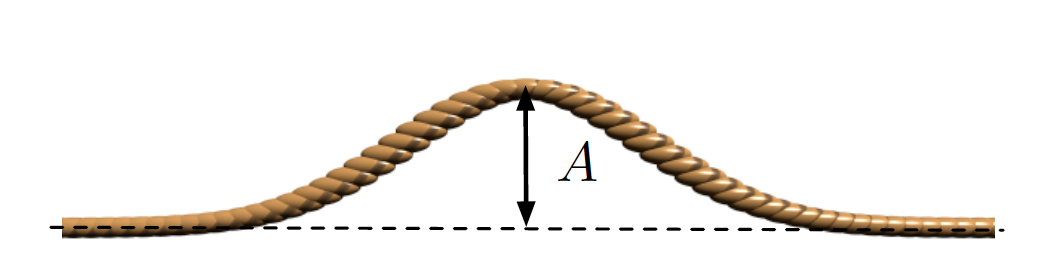
The location of the rope before and after the pulse passes is the equilibrium position . The maximum magnitude of the displacement of the pulse from equilibrium is called the amplitude , \(A\) . We define \(A\) to be always positive even if the displacement is below rather than above the equilibrium dashed line shown in the figure above. The amplitude depends on the source, or the amount of energy transferred to the medium by an external object, such as your hand shacking the rope. Another example of a pulse-type wave is the example of a rock thrown into a pond. In this example the rock produces many ripples, but they are a pulse wave because there are a finite amount of ripples with a well-defined beginning and end.
A Periodic Wave
Repeating waves can have many different shapes. One of the simplest to work with looks like a sine or a cosine function. Such waves are called harmonic or sinusoidal waves. They are generated by oscillators moving in simple harmonic motion, like the spring-mass system you studied in 7A. In other words, a harmonic wave can be modeled with motion of each particle in the medium described by spring-mass oscillator. If you hold one end of a rope and jiggle it up and down in simple harmonic motion, moving your hand in a periodic repeating manner, you will generate harmonic waves. If you were to take a picture of the wave, it might look like the figure below.
Figure 8.1.4: Periodic Wave
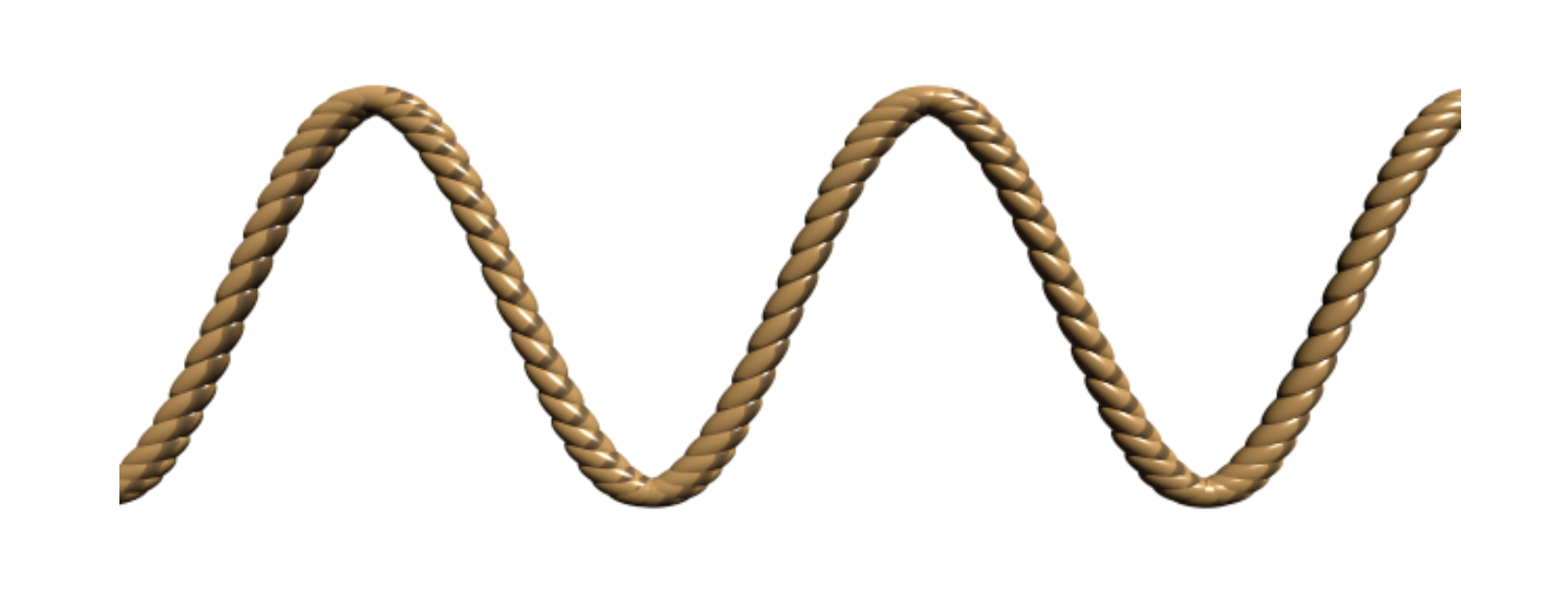
When you "take a picture" of a wave you capture its shape at some instance of time, which we call the snapshot of the wave. This can be represented graphically as shown below. Here \(x\) is the distance along the rope, and the displacement , \(y\) , represents how far the rope is displaced from equilibrium.
Figure 8.1.5: Graphical Representation of a Harmonic Wave at a Fixed Time ("Snapshot")
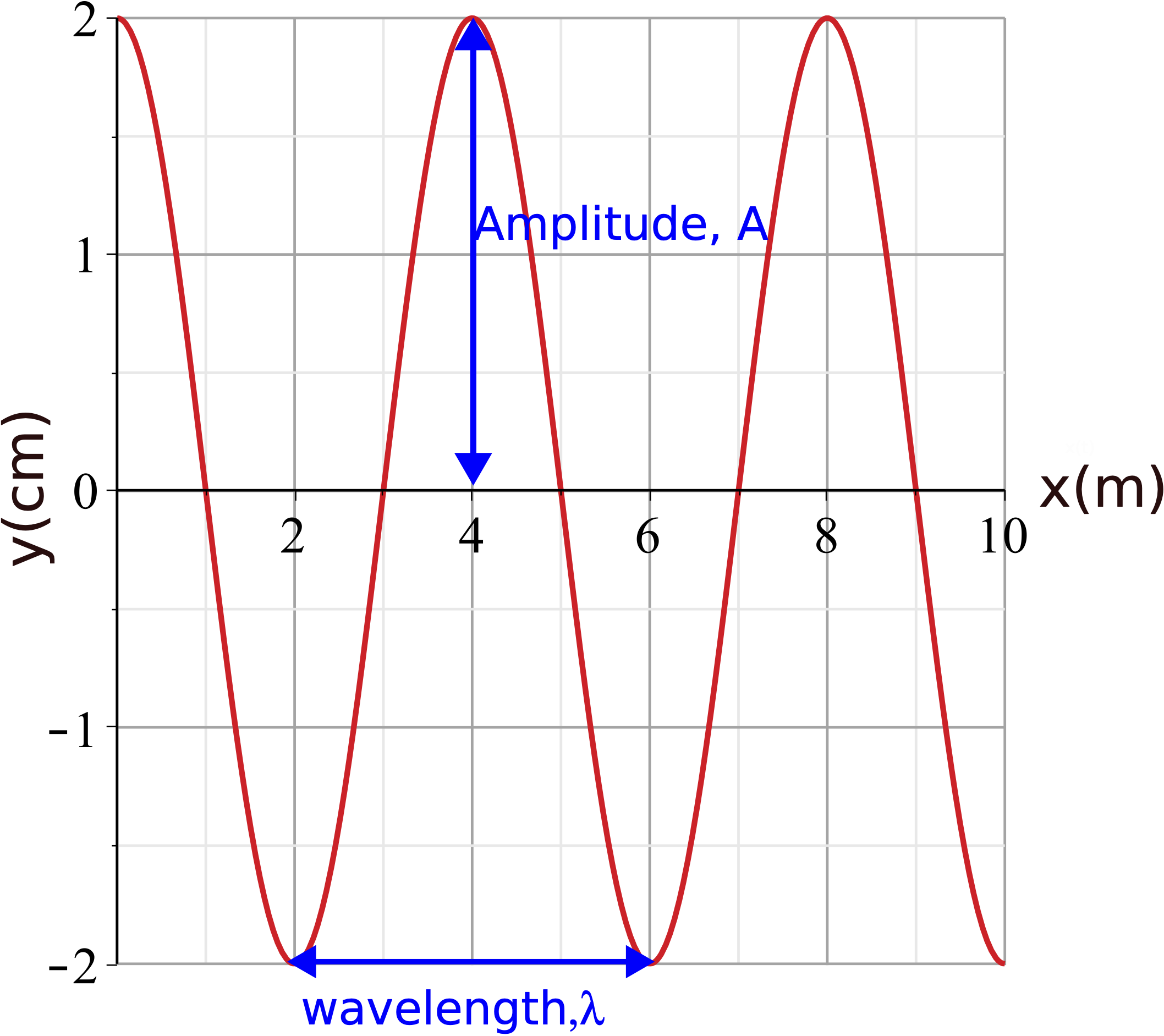
Like the wave pulse, a repeating wave has a well-defined equilibrium position and an amplitude, \(A\). In Figure 8.1.5 the equilibrium position is at y=0 cm, and the amplitude is 2 cm. Compared to a wave pulse, a repeating wave has two new parameters. The first is the wavelength , \(\lambda\) , which tells us the shortest distance (along the direction of wave motion) between identical parts of the wave. In other words, the wavelength represents the length of the spatial cycle of the wave as marked in Figure 8.1.5 above. In this figure \(\lambda= 4\) m.
The second new parameter is the period , \(T\) , the time it takes for the wave to look exactly the same. In other words, the wavelength tells us how the wave repeats in space , while the period tells us how the wave repeats in time . One period is the time it takes for the wave to move a distance of one wavelength, since it will look the same after one cycle. Oscillators in the medium go through one cycle during the time of one period, such as a spring-mass returning to its starting position. Pulse-type waves, which do not repeat, do not have periods or wavelengths. The wave period is determined by how often the source is disturbing the medium. If you were generating a harmonic wave on a rope, you can determine the period by measuring how long it takes for your hand to move up and back down.
Since the graph in Figure 8.1.5 shows the wave for a fixed time, so it gives us no information about the period which is time-dependent. To give a representation of the temporal characteristic of the wave, we need a new graph. Since there are two variables which describe the wave, space and time, in order to graph the wave changing with time we now need to represent it at a fixed position. If we were to paint a red dot on the rope at some fixed \(x\) value, and then plot the position of only that dot against time, we would find how that particular point moves in simple harmonic motion (see Figure 8.1.7 below). Thus, when representing the motion of the wave as a function of time, we are showing the harmonic oscillation of one specific particle in the medium which is at some fixed location in space. It takes the same amount of time for the "dot" to return to an initial position as it does for the whole wave to return to an initial configuration, as you can see in Figure 8.1.7 . An example of a displacement versus time graph is shown below.
Figure 8.1.6: Graphical Representation of a Harmonic Wave at a Fixed Position
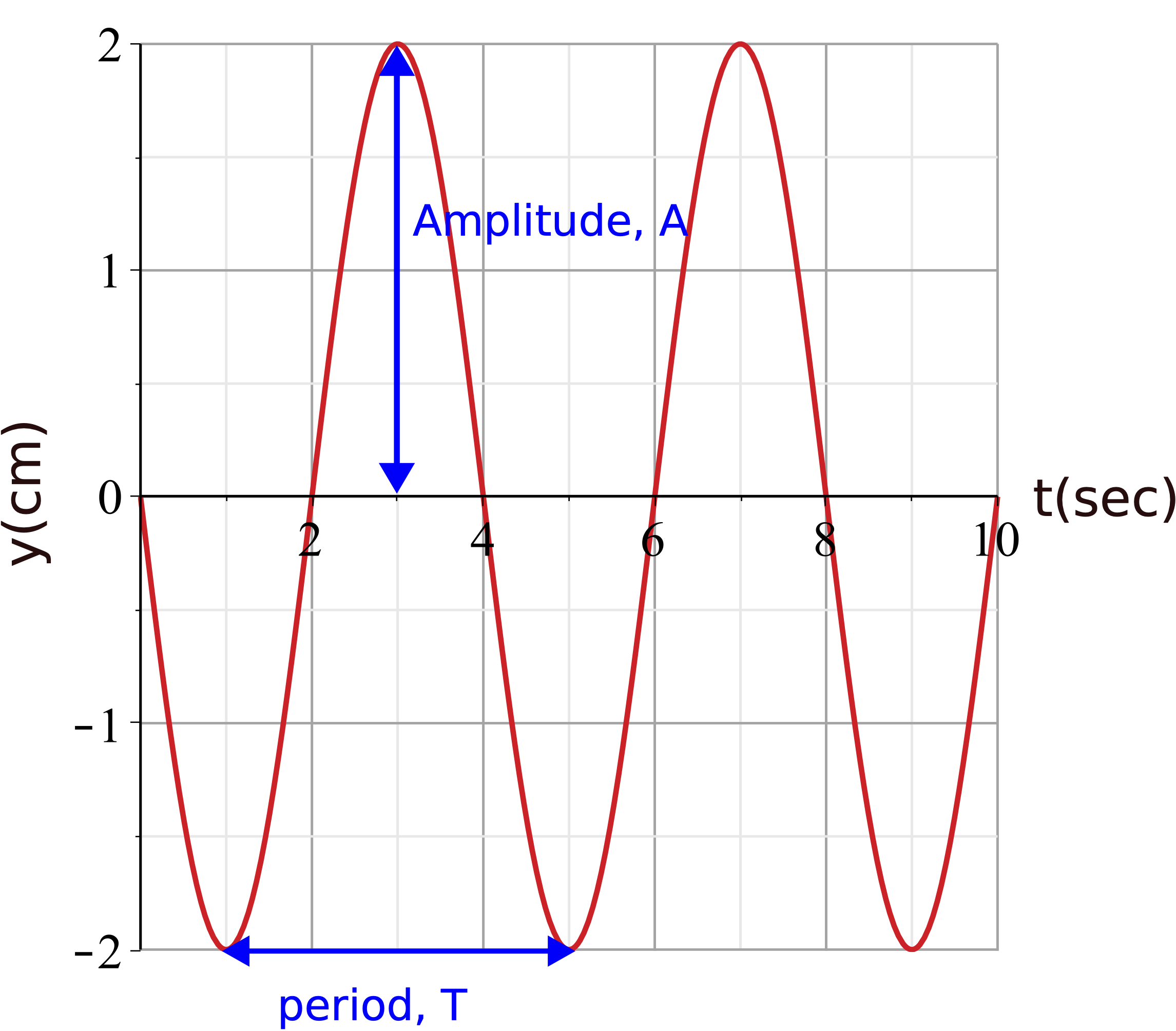
The period representing one temporal cycle is 4 seconds in the graph above. Assuming that figures 8.1.5 and 8.1.6 represent the same wave, the information they provide must be consistent. For example, both graphs show the same amplitude of 2 cm. The maximum displacement, known as the crest of the wave, is at 2 cm, the minimum displacement, the trough of the wave, is at -2 cm, and the midpoint between the crest and the trough is the equilibrium position, here at y=0 cm. In Figure 8.1.5 we see that the particle at position x=0 m is at a crest, position x=1 m is at equilibrium, and x=2 m is at a trough. Let us assume that Figure 8.1.5 is a snapshot at t=0 sec. Since Figure 8.1.6 shows t=0 sec at equilibrium, that means that the position it represents has to be at equilibrium in Figure 8.1.5 . Thus, possible positions for the time-dependent plot in Figure 8.1.6 could be x=1, 3, 5, etc, where the displacement is at equilibrium in Figure 8.1.5 .
The period is the amount of time for one cycle. Another useful measure of the periodicity of a wave is the number of cycles that fit in some unit of time, known as the frequency . Frequency is the reciprocal of the period:
\[T = \dfrac{1}{f}\label{Tf}\]
Typically, period is measured in seconds and frequency is measured in units called Hertz, Hz (1 Hz = 1 s -1 ). The period is the time between the arrival of adjacent crests of the wave, while the frequency is the number of crests that pass by per second. This means the red dot in Figure 8.1.7 completes an \(f\) number of up-and-down cycles every second. Equivalently, if you focus on a specific position in space, wave will return to the same configuration an \(f\) number of times every second.
Dimensionality
The examples seen above are called one-dimensional waves because the wave only travels in one direction. We arbitrarily called that direction the \(x\)-axis. If a wave spreads out on a surface instead, then it is a two-dimensional wave . For example, ripples on the surface of a pond represent a two-dimensional water wave. A wave that spreads outward in all directions is a three-dimensional wave . Examples of three-dimensional waves are typical sound and light waves.
An important distinction between these waves is that the amplitude \(A\) of the waves is only constant for one-dimensional waves. Two-dimensional and three-dimensional waves have amplitudes that depend on the distance from the source of the disturbance. Ripples in a pond become smaller as they spread out, and the brightness (amplitude of light) and loudness (amplitude of sound) of light and sound waves, respectively, decreases with distance away from the sources. This is a consequence of conservation of energy, as a wave propagates outward in multiple dimensions, the energy it carries must be spread over a region of increasing size.
Polarization
Material waves and electromagnetic waves have a characteristic called polarization . The polarization relates the direction of wave displacement to the direction of wave motion. For material waves, we are going to define two types of polarization:
- Transverse Polarization : A material wave is transverse if the displacement from equilibrium is perpendicular to the direction the wave is traveling. Below is a transverse wave since the wave is traveling to the right, while the oscillations in the medium are vertical, or perpendicular to the motion. The "red dot" represents one oscillator moving up and down periodically as the wave propagates to the right through the medium. An example of a transverse wave, is a wave generated on a rope or string as in Figure 8.1.4 . A surface water wave is another example, since the oscillations of the water particles are in the vertical direction while the wave propagates on the two-dimensional plane of the water surface.
Figure 8.1.7: Transverse Wave
- Longitudinal Polarization : A material wave is longitudinal if the medium displacement from equilibrium is in the same direction that the wave is traveling. In most examples of longitudinal waves that we explore, this displacement occurs as periodic compressions (region of more dense medium) and rarefactions (regions of less dense medium) of the material. The figure below shows an example of a longitudinal wave on a spring (or slinky). The most common example of longitudinal waves are sound waves, which we will discuss in more detail in a later section.
Figure 8.1.7: Longitudinal Wave
Although, transverse waves resemble physically the plots that we drew in figures 8.1.5 and 8.1.6 , we represent harmonic longitudinal wave exactly the same way using sinusoidal functions. The displacement on the y-axis in figures 8.1.5 and 8.1.6 does not need to represent the physical depiction of the wave, but rather it shows the distance from equilibrium in the medium. As in the transverse wave, the red dot in the longitudinal waves oscillates sinusoidally. Locations of compressions represent crests while rarefaction represent troughs.
The wave speed , \(v_{\text{wave}}\) , is the speed at which the disturbance propagates through the medium. One way of thinking about the wave speed is that it is the speed someone who was riding the wave on a surfboard would travel. It is not the speed of the oscillations of the individual particles making up the medium. In fact, from 7A we know that the speed of a harmonic oscillator (spring-mass) is not a constant, but changes with distance from equilibrium. The speed is maximum at equilibrium, and decreases as the oscillator gets further away from equilibrium, reaching zero at the maximum distance from equilibrium.
To a good approximation \(v_{\text{wave}}\) depends only on properties of the medium, not on wave amplitude or frequency. For large waves, or for waves with extreme frequencies, this approximation breaks down. For now, we simplify our discussion by ignoring dependence of wave speed on amplitude (we do not work with big wave in 7C). We will also ignore dependence of speed on frequency, until we discuss refraction of light waves in a later chapter.
The definition of speed from 7B can be written as:
\[\textrm{speed} = \dfrac{\textrm{distance travelled}}{\textrm{time spent}}\]
One period is the shortest amount of time before the wave looks exactly the same. When the wave looks exactly the same again, it has moved a distance of one wavelength by definition. Since it take the wave a time of one period to travel a distance of one wavelength, the wave speed can be written as:
\[v_{\text{wave}} = \dfrac{\lambda}{T}=\lambda f\]
The second equality above uses the definition of frequency from Equation \ref{Tf}. As an example of how the medium determines the wave speed we can look at a material wave on a stretched (characterized by tension) medium, such as a rope or a rubber hose. Both transverse waves and longitudinal waves are possible on a stretched strings or ropes. The speed, \(v_{\text{wave}}\), of transverse waves on a stretched string depends on the properties of the string that affect its elasticity and its internal properties. For a string that is thin compared to its length, the relation between the wave speed to the string properties is given by:
\[v_{\text{wave}} = \sqrt{\dfrac{T}{\mu}}\]
where \(T\) is the tension in the string and \(\mu\) is its mass density, mass per unit length (\(\mu=m/L)\). We can make some sense of the formula by considering the diagram in Figure 8.1.1 . The tension is (roughly) the force that one piece of string exerts on another. The tighter the string the higher the tension. As we learned, a material wave is a disturbance that propagates by one piece of the medium exerting a force on its neighbors, it is logical that an increase in tension results in an increase in the wave speed. Stronger forces will lead to greater acceleration. When the string is particularly heavy, the forces between pieces of the string result in less acceleration, so it also intuitive that as \(\mu\) increases the wave speed decreases. The ability to control the wave speed is critical for stringed instruments like a guitar or a violin, which is why they have tuning knobs at one end (to control the tension), and the strings are of different mass (for different values of \(\mu\)). We will discuss string instruments in more detail when we cover standing waves in later sections.

IMAGES
VIDEO
COMMENTS
Describing a Wave. A wave can be described as a disturbance in a medium that travels transferring momentum and energy without any net motion of the medium. A wave in which the positions of maximum and minimum amplitude travel through the medium is known as a travelling wave. To better understand a wave, let us think of the disturbance caused ...
A traveling wave in a medium is a disturbance of the medium that propagates through it, in a definite direction and with a definite velocity. By a "disturbance" we typically mean a displacement of the parts that make up the medium, away from their rest or equilibrium position.
Figure 16.3 An idealized surface water wave passes under a seagull that bobs up and down in simple harmonic motion. The wave has a wavelength λ λ, which is the distance between adjacent identical parts of the wave.The amplitude A of the wave is the maximum displacement of the wave from the equilibrium position, which is indicated by the dotted line. . In this example, the medium moves up and ...
The magnitude of the wave velocity is the distance the wave travels in a given time, which is one wavelength in the time of one period, and the wave speed is the magnitude of wave velocity. In equation form, this is. v = λ T = λf. (16.2.1) (16.2.1) v = λ T = λ f.
4.2 - Travelling waves. Travelling waves; A travelling wave is a continuous disturbance in a medium characterized by repeating oscillations. For example: A rope that is flicked up and down continuously creates a repeating disturbance similar to the shape of a sine/cosine wave. Energy is transferred by waves. Matter is not transferred by waves.
A traveling wave in a linear system is a pair of standing waves put together with a special phase relation. We show how traveling waves can be produced in finite systems by appropriate forced oscillations. We then go on to discuss the force and power required to produce a traveling wave on a string, and introduce the useful idea of "impedance
Traveling Waves vs. Standing Waves. A mechanical wave is a disturbance that is created by a vibrating object and subsequently travels through a medium from one location to another, transporting energy as it moves. The mechanism by which a mechanical wave propagates itself through a medium involves particle interaction; one particle applies a ...
The magnitude of the wave velocity is the distance the wave travels in a given time, which is one wavelength in the time of one period, and the wave speed is the magnitude of wave velocity. In equation form, this is. v= λ T = λf. v = λ T = λ f. This fundamental relationship holds for all types of waves.
Introduction to waves. Transverse and longitudinal waves are two types of mechanical waves, which involve the transfer of energy through a medium (e.g. water, air, a solid). Learn about transverse and longitudinal waves through the examples of a shaken rope and a sound wave.
A wave is a repeating disturbance that travels through matter or space transferring only energy. Below is a model of a wave. A wave's crest is its highest point, and its trough is its lowest point. A wave's amplitude is the maximum distance (positive or negative) a wave reaches from its rest position. Wavelength is the distance between the ...
Waves can be classified according to what they appear to be doing. traveling waves …are waves that appear to be propagating. This might seem like a distinction made by the Department of Redundancy Department, since propagation is a key part of the definition of a wave, but there actually are waves that do not appear to be going anywhere.
A wave is a disturbance that propagates from its source, repeating itself periodically, and is typically associated with simple harmonic motion. Mechanical waves are governed by Newton's laws and require a medium to travel. A medium is a substance in which a mechanical wave propagates, and the medium produces an elastic restoring force when it ...
It is generated through a solid object like a stretched rope. Trampoline is the best example to understand this wave. Longitudinal Waves - in this type of travelling wave the motion of the wave-particle is in the same direction as the propagation of the wave. In simple words, the movement of the particles is parallel to the motion of the energy.
Likewise, a traveling wave is a combination of standing waves. For example, These relations are important because they show that the relation between and, the dispersion relation, is just the same for traveling waves as for standing waves! A wave is a wave, whether traveling or standing. Indeed, we can go back and forth using (8.7) and (8.8).
16.1: Travelling Waves. A wave is a disturbance that propagates from its source, repeating itself periodically, and is typically associated with simple harmonic motion. Mechanical waves are governed by Newton's laws and require a medium to travel. A medium is a substance in which a mechanical wave propagates, and the medium produces an elastic ...
Ebiuwa. 4 months ago. For people who don't understand the concept of Transmission and Refraction: Medium: An object that a wave can travel through. Transmission: When a wave (like light) travels through a medium. Light waves travel through EMs, or electromagnetic fields. Mechanical waves, like water, can travel through physical mediums, like a ...
wave, propagation of disturbances from place to place in a regular and organized way. Most familiar are surface waves that travel on water, but sound, light, and the motion of subatomic particles all exhibit wavelike properties. In the simplest waves, the disturbance oscillates periodically ( see periodic motion) with a fixed frequency and ...
0:00 - Intro0:14 - Traveling waves & transverse waves defined6:18 - The wave equation10:06 - Graphing waves12:46 - Longitudinal waves
Travelling waves are the kind of waves where the position of the particles shifts as the wave advances. The wave's maximum and minimum amplitudes pass across the medium, and its constituent particles oscillate in accordance with the wave's progression. Progressive waves are another name for moving waves.
When a wave travels through a medium-i.e., air, water, etc., or the standard reference medium (vacuum)-it does so at a given speed: this is called the speed of propagation. The speed at which the wave propagates is denoted and can be found using the following formula: v = fλ (1.5.1) (1.5.1) v = f λ.
The P-wave gets progressively farther ahead of the S-wave as they travel through Earth's crust. For that reason, the time difference between the P- and S-waves is used to determine the distance to their source, the epicenter of the earthquake. We know from seismic waves produced by earthquakes that parts of the interior of Earth are liquid.
Since it take the wave a time of one period to travel a distance of one wavelength, the wave speed can be written as: vwave = λ T = λf (8.1.3) (8.1.3) v wave = λ T = λ f. The second equality above uses the definition of frequency from Equation 8.1.1 8.1.1.