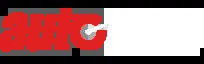
- Limited 6.0L 4x4

Limited 6.0L 4x4 2003 Ford Excursion Crash Test Ratings
- Safety Features
- Crash Rating
More Excursion Information
- All Model Years
- Excursion News
- Book Values
- Ford Dealers
2003 Ford Excursion trims (30)
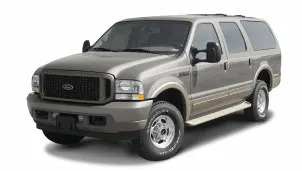
(XLT 5.4L Value) 4x2
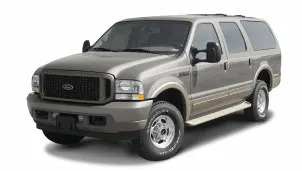
(XLT 6.8L Value) 4x2
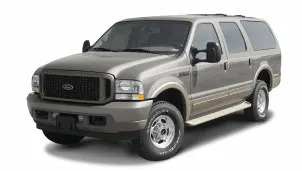
(XLT 5.4L Premium) 4x2
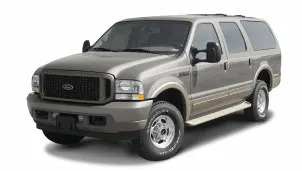
(XLT 6.8L Premium) 4x2
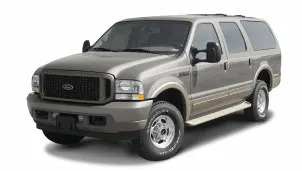
(XLT 5.4L Value) 4x4
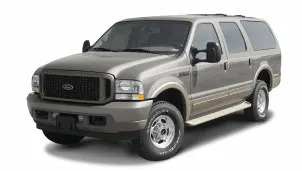
(XLT 6.8L Value) 4x4
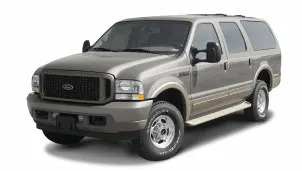
(Eddie Bauer 5.4L) 4x2
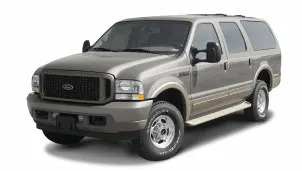
(XLT 7.3L Value) 4x2
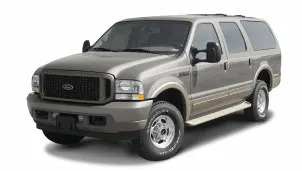
(XLT 6.8L Premium) 4x4
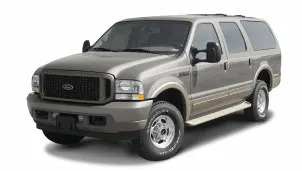
(XLT 5.4L Premium) 4x4
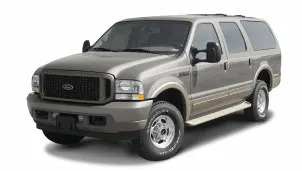
(Eddie Bauer 6.8L) 4x2
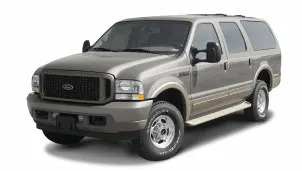
(XLT 6.0L Value) 4x2
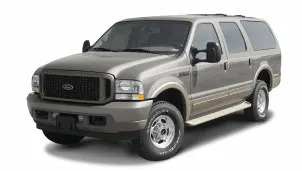
(Limited 5.4L) 4x2
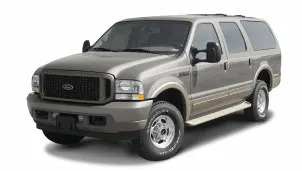
(XLT 7.3L Premium) 4x2
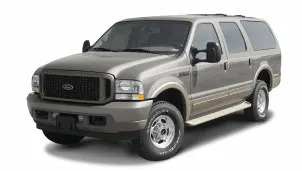
(Limited 6.8L) 4x2
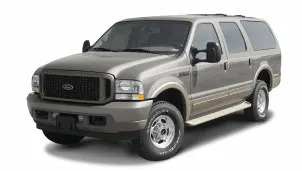
(XLT 6.0L Premium) 4x2
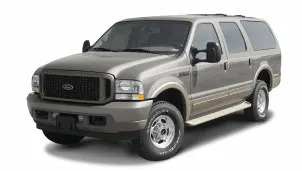
(XLT 7.3L Value) 4x4
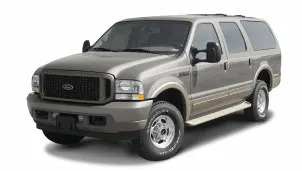
(Eddie Bauer 6.8L) 4x4
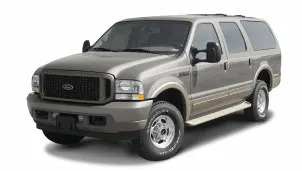
(XLT 6.0L Value) 4x4
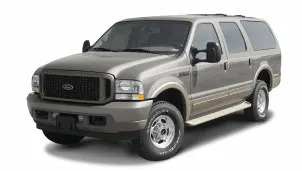
(XLT 7.3L Premium) 4x4
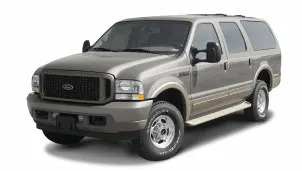
(Eddie Bauer 7.3L) 4x2
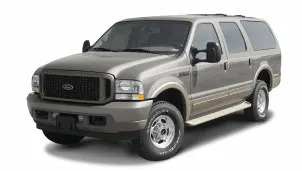
(Limited 6.8L) 4x4
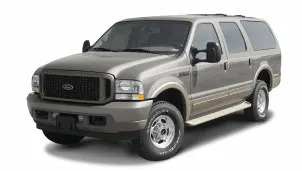
(XLT 6.0L Premium) 4x4
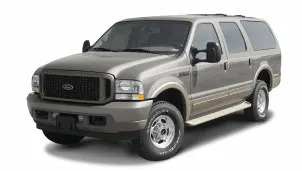
(Eddie Bauer 6.0L) 4x2
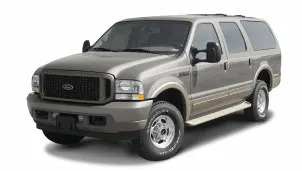
(Limited 7.3L) 4x2
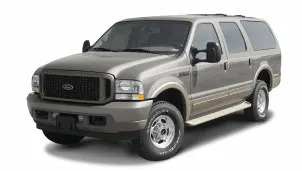
(Limited 6.0L) 4x2
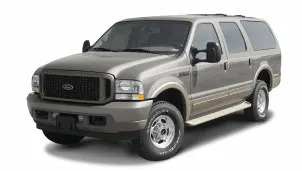
(Eddie Bauer 7.3L) 4x4
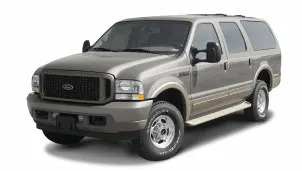
(Eddie Bauer 6.0L) 4x4
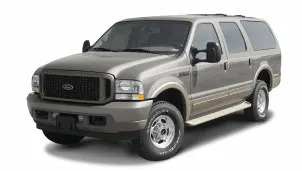
(Limited 7.3L) 4x4
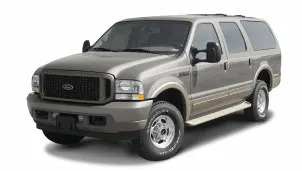
(Limited 6.0L) 4x4
Popular vehicles, featured makes, product guides.
- The Best Electric Bikes
- The Best Car Covers
- The Best Portable Air Compressors
- The Best Car GPS Trackers
Just One More Thing…
CarComplaints.com : Car complaints, car problems and defect information
Latest news.
- Apr 7: Mallory Harcourt v. Tesla
- Apr 6: Kia Hydraulic Clutch Actuator Recall Failed, Alleges Lawsuit
- My Account |
- Investigations
- Search Complaints
- Update Carcomplaints
- Add Complaints
- Recent Trends
- Best Vehicles
- Worst Vehicles
- Worst Complaints
Donate your FORD EXCURSION!
Is it time to move on? Support NPR & the programs you love. Donate your old car in minutes through the CarTalk Vehicle Donation Program.
- Ford »
- Excursion »
- 2002 »
- Lamborghini
- Mercedes-Benz
- Bronco Sport
- C-Max Energi
- C-Max Hybrid
- Country Squire
- Crown Victoria
- Escape Hybrid
- Explorer Sport Trac
- F-150 Lightning
- Fiesta Encore
- Five Hundred
- Focus Electric
- Fusion Energi
- Fusion Hybrid
- Fusion Plug-In Hybrid
- Maverick Hybrid
- Mustang Mach-E
- Thunderbird
- Tourneo Custom Van
- Transit 250
- Transit Connect

2002 Ford Excursion
Crash test safety ratings.
- Compare Excursion Years
- Complaints 154
- Crash Tests 1
- Excursion Recalls 5
- Defect Investigations 4

NCAP 5-Star Crash Test & Rollover Safety Ratings
Iihs crash test & rollover safety ratings.
The IIHS has not conducted crash tests for the 2002 Excursion yet.
Ratings only apply to the model and body or seat type tested. The date of manufacture and optional safety equipment can affect results. For full details see www.iihs.org .
Search for Car Problems
Example: "Bad Brakes", "Toyota Recall", etc.
Add Your Complaint
Bump the Excursion problem graphs up another notch. Get answers and make your voice heard!
Become a Fan & Spread the Word
NHTSA’s Crash Test Dummies
Meet NHTSA’s family of crash test dummies currently in service. The diverse group of dummies helps us understand and measure the human body’s movement during a crash, and see how it fares with various vehicle safety features.
A lot of research goes into these dummies before they are put into use. Each of us differs in size and weight, so each crash test dummy is designed differently too. NHTSA’s family of dummies representation ranges from newborn infant to 6-year-old children to small females and average males. We're always looking to enhance their abilities, and in recent years advanced biomechanics research and measurement technologies have helped to improve crash dummy development.
What we learn from these dummies in our comprehensive crash tests helps to improve vehicle safety — saving more lives.
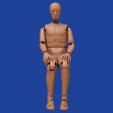
50th Percentile Adult Male
Hybrid III
5’ 9” - height represented
171 lbs - actual weight
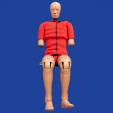
50th Percentile Adult Male
ES-2re
160 lbs - actual weight
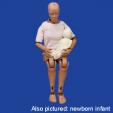
5th Percentile Adult Female
4’ 11” - height represented
108 lbs - actual weight
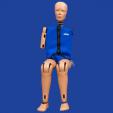
Small Adult Female
SID-IIsD
97 lbs - actual weight
10-Year-Old Child
4’ 8” - height represented
78 lbs - actual weight
6–Year-Old Child
3’ 11” - height represented
52 lbs - actual weight
6-Year-Old Weighted Child
62 lbs - actual weight
3-Year-Old Child
3’ 1” - height represented
36 lbs - actual weight
12-Month-Old Infant
22 lbs - actual weight
Newborn Infant
7.6 lbs - actual weight
When people think of crash test dummies, they often associate it with: "You Could Learn a Lot From a Dummy.” This famous tagline was the foundation of a popular NHTSA safety campaign urging people to wear their seat belts. Vince and Larry, the loveable crash test dummies, encouraged seat belt use through TV, print, and radio ads. Vince and Larry have been inducted into the Smithsonian Institution's Museum of American History as part of an exhibit on the evolution of automobile safety – along with authentic crash test dummies.
NHTSA's 5-Star Safety Ratings

An official website of the United States government
The .gov means it’s official. Federal government websites often end in .gov or .mil. Before sharing sensitive information, make sure you’re on a federal government site.
The site is secure. The https:// ensures that you are connecting to the official website and that any information you provide is encrypted and transmitted securely.
- Publications
- Account settings
Preview improvements coming to the PMC website in October 2024. Learn More or Try it out now .
- Advanced Search
- Journal List
- Annu Proc Assoc Adv Automot Med
Head Excursion of Restrained Human Volunteers and Hybrid III Dummies in Steady State Rollover Tests
Seatbelts provide substantial benefits in rollover crashes, yet occupants still receive head and neck injuries from contacting the vehicle roof interior when the roof exterior strikes the ground. Prior research has evaluated rollover restraint performance utilizing anthropomorphic test devices (dummies), but little dynamic testing has been done with human volunteers to learn how they move during rollovers.
In this study, the vertical excursion of the head of restrained dummies and human subjects was measured in a vehicle being rotated about its longitudinal roll axis at roll rates from 180-to-360 deg/sec and under static inversion conditions. The vehicle’s restraint design was the commonly used 3-point seatbelt with continuous loop webbing and a sliding latch plate. This paper presents an analysis of the observed occupant motion and provides a comparison of dummy and human motion under similar test conditions. Thirty-five tests (eighteen static and seventeen dynamic) were completed using two different sizes of dummies and human subjects in both near and far-side roll directions.
The research indicates that far-side rollovers cause the restrained test subjects to have greater head excursion than near-side rollovers, and that static inversion testing underestimates head excursion for far-side occupants. Human vertical head excursion of up to 200 mm was found at a roll rate of 220 deg/sec. Humans exhibit greater variability in head excursion in comparison to dummies. Transfer of seatbelt webbing through the latch plate did not correlate directly with differences in head excursion.
Census reporting of fatal crashes indicates that rollovers accounted for 19.1% of the fatal crashes in the United States in 1999, which is a high percentage when compared to the relative frequency of rollover crashes [ NHTSA, 2000 ]. Illustrating the benefits of seatbelts in rollover crashes, Digges et al. (1998) reviewed government rollover data from 1988–1996 and determined that 80.4% of the occupants who were seriously or fatally injured were unrestrained, and had a much greater likelihood of being injured or killed than belted occupants. Lateral rollover crashes, the most prevalent type of rollover event, are responsible for about 90% of the harm in rollovers [ Digges et al., 1991 ]. In lateral rollovers, the “near-side” (the leading side) occupants are exposed to a much different event than the “far-side” (the trailing side) occupants. Far-side occupants are exposed to greater rotational velocities and move higher relative to the ground, which can lead to more severe ground contacts [ Moffatt, 1975 ]. Parenteau et al. (2001) utilized statistical data and confirmed that far-side occupants were at a greater risk for serious injury in rollover crashes when compared to near-side occupants.
PREVIOUS VEHICLE AND LABORATORY TESTING
When evaluating rollover crash testing with restrained dummies, Bahling et al. (1990) , Moffatt et al. (1995) , Friedewald et al. (1996) and Hare et al. (2002) have demonstrated the restraining capabilities of seatbelt systems, but little scientific data has been available regarding the human response to rollover forces. Although crash dummies have been highly developed to provide biofidelic responses in certain crash conditions, the similarity between human and crash dummy response in a restrained rollover condition is less established.
Several researchers have investigated the excursion available to seatbelted crash dummies and human volunteers when inverted statically. Bahling et al. (1990) reported static inversions of three restrained human (50 th percentile male) volunteers in a 1983 Chevrolet Malibu, resulting in an average of 99 mm of head excursion. Arndt et al. (1995) also performed static inversions using lap belted volunteers and the 95 th percentile Hybrid III dummy to document head excursion with two lap belt angles and various lap belt tensions/slack analyzed. They found that less head excursion resulted when the lap belt was more vertically angled and was pretensioned. Herbst et al. (1996) , Friedman et al.(1996) , and Meyer et al. (2000) conducted several static and slow dynamic (100 deg/sec) tests with the human subjects in a seat that was rotated about the approximate center of gravity of the seated occupant. Meyer et al. (2000) compared production restraints to experimental pretensioned restraints and concluded excursion was reduced by 80% with the experimental systems. The pretensioners in those tests were activated before any roll angle was introduced. James et al. (1997) also performed static inversions, resulting in approximately 127 mm of head excursion for two human subjects in fourteen different vehicles. They did not note any significant differences in excursion between the two humans or the various production restraints. Ward et al. (2001) presented static inversion data for human subjects and Hybrid III dummies. They reported head vertical excursion measurements similar to the findings of other researchers for their tests with seatbelts utilizing a cinching latch plate. However, their sliding latch plate tests showed vertical excursions of the head to be about two to three times greater than in the cinching latch plate tests, a result that is not consistent with other researchers.
Several researchers measured the dynamic excursion of seatbelted subjects in seats that rotated about the approximate center of gravity of a rolling vehicle. Moffatt et al. (1997) conducted extensive inversion testing with Hybrid III dummy, human, and cadaver subjects in a laboratory fixture that rotated the seat about an axis inboard of the seat. In addition to static inversion testing, near-side dynamic testing at 280 deg/sec was also performed on the restrained dummies and cadaver. For this research, a production 3-point seatbelt without a retractor was utilized. The maximum head excursion for each test subject type is shown in Table 1 below for the various 3-point belt configurations. Pywell et al. (1997) conducted similar tests using the 50 th percentile Hybrid III dummy in a rotating fixture that utilized gravity to achieve a peak roll rate of 240 deg/sec. The various experimental restraint configurations had average vertical head excursions of 69 to 98 mm for non-pretensioned systems and 36 to 58 mm for pretensioned systems.
Moffatt et al. (1997) Maximum Head Excursion (mm) for Various 3-point Belts
METHODS OF THE CURRENT STUDY
Vehicle kinematics during rollover crashes may be considered to occur in two phases: the airborne phase and the impact phase. The airborne phase is when the vehicle is rotating in the air while not touching the ground, and the impact phase is when the vehicle strikes the ground. Most of the research above considers vertical excursion of a subject’s head under various simulations of the airborne phase of a rollover. Airborne-phase excursion research provides useful information because it helps explain the limits of motion that a seatbelted occupant might have in a vehicle before the ground impact occurs. The research that is the subject of this paper analyzes the vertical excursion of both restrained humans and similar-sized Hybrid III dummies in a complete vehicle that is rotated at realistic rollover rotational velocities. Understanding how restrained occupants move within a rotating vehicle provides insight into occupant motion in real rollover crashes. Vehicle-to-ground impacts, which are not addressed here, will cause additional relative movement between the vehicle and the occupant.
The test methodology chosen for the current study was controlled, multi-revolution rotation of a complete vehicle about a fixed, longitudinal axis through the vehicle center of mass. To compare the human and crash test dummy response, the research presented here measured the static and dynamic vertical head excursion of four human subjects and similar sized dummies in simulated airborne rollover conditions, including both far-side and near-side roll directions. Vehicle roll rates from 180 to 360 deg/sec were utilized for the dummy dynamic testing, while a roll rate of 220 deg/sec was utilized for the dynamic human testing. In addition, the movement of webbing through the latch plate was analyzed and compared to the head excursion results.
TEST FIXTURE
The test fixture was developed by Exponent, Inc. as part of its Controlled Rollover Impact System (CRIS) [ Cooper et al., 2001 ]. The complete CRIS system includes a moving fixture that drops a rotating vehicle in a controlled manner. A similar stationary laboratory fixture was developed for dynamically balancing a vehicle in preparation for a CRIS rollover test. It is this stationary system that was used for the current head excursion study.
The stationary, controlled roll fixture is depicted in Figure 2 . It consists of two heavy-duty stands mounted on a test bed. The stands support the front and rear ends of a test vehicle through adjustable pin mounts. The adjustability of the pin mounts is critical to the tuning of the roll axis position for a smooth, balanced roll. Several calibration rolls with small adjustments to the pin mounts are used to minimize the dynamic vehicle support loads prior to conducting actual tests on the fixture. A controller, roll rate sensor, and 0-deg index pulse provide the operator with the tools to drive the system at the desired speed for the desired number of rotations.

Test Fixture with 2001 Nissan Pathfinder Mounted for Rotation
TEST VEHICLE
The vehicle used for the current study was a production 2001 Nissan Pathfinder compact sport utility vehicle with front bucket seats. The door window frame, roof, and roof rail near the driver’s position were removed to eliminate any possibility of head strike and to enable full head excursion, unrestricted by the roof. To regain structural stiffness lost in removal of the roof section, structural bracing was added. Padding was added to the edges of the roof opening as an additional safety precaution.
Production driver bucket seat and restraint systems were used for all testing. The continuous loop, 3-point seatbelt system included a sliding latch plate, an adjustable upper anchorage (D-ring) and an emergency locking retractor (dual-locking type) mounted in the lower B-pillar. The sliding latch plate is currently the most common design choice of both domestic and import car manufacturers. An industry review shows this basic design utilized in 2003 model year vehicles for the following manufacturers: Dodge, Ford, Honda, Lincoln, Mercury, Volvo, Land Rover, Jaguar, Mazda, Ferrari, Maserati, Lamborghini, Bentley, Jeep, Mitsubishi, Subaru, Porsche, Hyundai, Audi, BMW, Lexus, Volkswagen, Acura, Chrysler, Toyota, Kia, Suzuki, Isuzu, Chevrolet, Buick, Oldsmobile, Pontiac, Hummer, Cadillac, Saturn, and GMC.
The Hybrid III dummy was first developed primarily for use in frontal impact testing, and as such, its body stiffness was designed for biofidelity under high acceleration events. In the airborne phase of rollovers, however, the accelerations are relatively low, so this dummy is not ideally suited for evaluation of all body movements. One of the goals of this study was to better understand how dummies and human subjects of similar stature differ in head excursion and overall kinematics under these same test conditions.
The two occupant sizes selected were the 50 th percentile adult male and the 5 th percentile adult female. One Hybrid III dummy of each size and two human subjects of each size were tested. The Hybrid III dummy utilized a pedestrian pelvis because the increased hip mobility was anticipated to offer more realistic dummy kinematics than a seated pelvis under these test conditions. For comparison, one dummy test was run with the seated pelvis. Two approximately 50 th percentile male subjects and two approximately 5 th percentile female subjects participated in the human subject test series. The human subjects were professional stunt people in excellent health and fitness, which is likely to be somewhat atypical of the average motoring public. The sizes of the human subjects are provided along with their dummy counterparts in Table 3 .
Occupant Sizes
HUMAN SUBJECT TESTING ISSUES
For the human testing, great care was taken to ensure the safety of the test subjects. The project team researched published literature and relied on prior laboratory test experience of an employee of Exponent who, coincidentally, had tested both himself and others to measure the physiological limits of vertical accelerations on human volunteers in simulated aircraft experiments. Of primary concern was the effect of “negative-z” acceleration on the pulmonary system. While seated and restrained in the rotating vehicle, a driver occupant experiences both tangential (along the path) and normal (directed toward the axis) acceleration. For a constant angular velocity, the tangential acceleration is zero because the amplitude of the tangential velocity is constant. However, the direction of the tangential velocity is changing, resulting in a non-zero acceleration directed toward the fixed rotational axis of the vehicle (normal acceleration). During vehicle rotation, the occupant’s body tends to lean outboard, resulting in the spine becoming generally aligned with the normal acceleration vector, which is approximately directed from the head toward the tailbone. In biomechanics terms, this direction is referred to as the negative-z axis of the human body. (Note that according to SAE dummy sign convention, this is considered the positive-z direction.) The greater the angular velocity, the greater the negative-z acceleration on the body, according to the equation:
where a z = normal acceleration (cm/sec 2 )
ω = angular velocity (radians/sec)
r = radius of particle from axis (cm)
An early indicator of the human body’s physiological response to negative-z acceleration is slowing of the heart rate as a result of the carotid baroreceptors sensing an increase in blood pressure. Further exposure can result in petechial hemorrhages around the eyes or on the sclera. The experiences of professional figure skaters during extreme spinning moves was one source of data that was considered when setting limits of exposure for the current study.
The drive system of the CRIS stationary roll fixture enabled the vehicle to spin up to the desired constant rotation rate in about three revolutions. The braking system enabled the vehicle motion to be arrested in about two revolutions. Three to four steady state revolutions, at the desired roll rate, were considered adequate for data collection. For the range of targeted roll rates under consideration (180 to 360 deg/sec), the total duration of a dynamic test would be about 20 seconds, with only about 3 seconds at the steady state rate. Given this duration, a negative-z acceleration limit for the subject’s head of –3 g was established. Results of the dummy test series indicated that this acceleration limit would safely enable human subject testing to be conducted at roll rates up to 240 deg/sec. For an added measure of safety and to provide direct comparison with data already collected in the dummy test series, 220 deg/sec was chosen as the targeted roll rate for the human test series.
A detailed test protocol was prepared that included the rationale for establishing the 240 deg/sec limit. The protocol was reviewed by an Exponent Human Testing Review Board, which was independent of this study, comprised of a Ph.D. in Experimental Psychology, a Ph.D. in Kinesiology, an M.S. in Mechanical Engineering (Biomechanics), and an M.D. who is currently a practicing pediatrician. The project team worked with the review board to provide a carefully executed human subject study that comprised the following:
- The human subjects were provided a copy of the protocol reviewed by the board so that they would be well informed prior to agreeing to participate.
- Each subject completed a medical questionnaire developed by the project team and review board. The subjects were accepted for the study on the basis of review and acceptance of their completed questionnaires by the Exponent M.D.
- The subjects were given physical examinations by a medical doctor the day before their participation. He found them to have no medical restrictions.
- A licensed paramedic participated in the testing, monitoring the human subjects’ vital signs before and after each dynamic test. They showed no signs of discomfort or negative health effects as a result of the testing.
- The human subjects watched the vehicle being rolled on the fixture prior to their own participation. They watched video tape of the dummy tests to see the motion of the dummies, and they were allowed to watch each other being tested.
- A brake was incorporated into the test fixture to reduce the number of coast down rotations following data collection.

Supplemental Harness System
- An abort switch was provided for the subject to hold. Release of thumb pressure on the switch would result in automatic shutdown of the system and activation of the pneumatic cylinder on the supplemental harness system. (None of the subjects opted to abort any test early.)
DATA COLLECTION
Data was collected from instrumentation and real time video recordings taken during the tests, as outlined in Table 5 :
Instrumentation and Camera Views
Post-test analysis of footage from cameras 3 and 5 yielded head excursion and belt motion information. The webbing was marked with lines spaced at 2.54 cm at the latch plate, to enable measurement of the webbing movement through the latch plate during each test. A vertical wand with photographic markings spaced at 2.54 cm was attached to the occupant’s head to facilitate the excursion measurements. Figure 6 shows one of the subjects wearing the head wand and yellow supplemental restraint harness prior to a test.

Human Test Subject with Head Wand and Supplemental Restraint
TEST MATRIX AND TEST PROCEDURES
The seat bottom was positioned at the mid-track fore-aft location, with the seatback adjusted to the design position for the comparison static and dynamic testing of the human subjects and both sized dummies. For the 50 th percentile male subjects the D-ring was positioned at the second from top position and for the 5 th percentile female subjects it was placed in the lowest position.
All of the subjects were normally seated wearing the production restraint system. For the dummies, the hands were taped together to facilitate camera coverage. Prior to the far-side roll direction tests, the shoulder belt was moved distally from the clavicle area onto the upper arm to prevent it from becoming entrapped in the shoulder joint. The matrix of tests with this standard seat position is presented in Table 7 .
Test Matrix
For the static testing, the vehicle was slowly rotated manually to 180 degrees. Once inverted, the dummies were shaken to allow them to settle into the restraint system. The human subjects settled themselves. The head excursion was determined from post-test review of the video footage by evaluating the difference in the amount the head wand protruded past the roof for the upright and inverted positions.
In addition to the seat mid-position testing, the effects of seat position were studied for the 5 th percentile female surrogate by varying the seat base and seatback positions. This testing was conducted under two conditions: with the seatback in its most-upright adjustment and the seat base mid-track, and with the seatback at the design position and the seat base set full forward.
For the dynamic testing, the rotation started with the fixture upright and the subject normally seated. The vehicle was then rotated at incremental roll velocities between 180 deg/sec and 360 deg/sec. This range of rotational speeds is not uncommon during rollover crashes. In rollover tests by Hughes et al. (2002) , the peak roll velocities for the first roll were reported to be 270 – 390 deg/sec, and Orlowski (1985) reported early roll rates around 310 – 400 deg/sec, with later roll velocities up to 500 deg/sec.
STATIC INVERSION TESTING
All dummies and human subjects were first tested in two static inversion tests: one rotating to the near-side and one to the far-side. The seatbelt retractor was allowed to lock normally as the vehicle was slowly rotated. The results of the static inversion tests are shown in Figure 8 . The additional seating positions utilized for the 5 th percentile surrogate evaluation are shown in Appendix A.

Dummy and Human Head Excursions in Static Tests.
As seen in Figure 8 , there was little variation in the static head excursion between the dummies and humans. The 5 th percentile female dummies averaged 130 mm excursion, compared to the 5 th percentile human subject average of 127 mm. The 50 th percentile male dummies averaged 124 mm compared to 122 mm for the 50 th percentile humans. The average head excursion for far-side static roll (129 mm) and the near-side static roll (122 mm) were similar.
DYNAMIC HEAD EXCURSION TESTING
The dummy and human occupants were tested in both near and far-side dynamic rotations. The dummies were spun up to 360 deg/sec and the humans to 220 deg/sec. Shown below in Figure 9 is the dynamic head excursion for all of the dummies and the humans at the 220 deg/sec rotation rate, including all seating positions for the 5 th percentile female dummy. For the dummies, as the rotation rate was increased from 220 deg/sec to 360 deg/sec, no substantial increase in the head excursion was observed.

Head Excursion of Dummies and Humans in All Dynamic Tests (220 deg/sec).
There are a number of interesting observations comparing data from Figures 8 and and9. 9 . First, there tended to be more excursion in the dynamic far side tests than in the static tests, but less excursion in the dynamic near side tests than in the static tests. Secondly, in the dynamic test series, only one near-side roll test, a 50 th percentile dummy, exceeded the lowest head excursion measured for all far-side roll tests.
COMPARISON OF HYBRID III DUMMIES TO HUMANS
Differences between the average dynamic vertical excursion of human and dummy subjects were minimal. The average vertical head excursion for the four different comparisons were: dummy in near-side roll = 108 mm, human in near-side roll = 111 mm, dummy in far-side roll = 188 mm, human in far-side roll = 165 mm. Figures 10 and and11 11 are two still images taken from the onboard video at the point of maximum vertical excursion for the 50th percentile male and dummy subjects. There were some differences between the kinematics of the humans and the dummies primarily because the dummies appear to be stiffer and have less lateral compliance. Also, the human response between subjects was widely varied. For example, one of the 50 th percentile human males had approximately 75 mm more vertical head excursion in the far-side dynamic test than the other. There was also a large difference in vertical excursion between the two 5 th percentile female subjects in the far-side dynamic tests. In each instance, the subject with more excursion had the shoulder belt farther off the shoulder than his similar sized counterpart.

50 th Percentile Male Dummy Head at Maximum Excursion

50 th Percentile Male Human Head at Maximum Excursion
COMPARISON OF DUMMY SEATED VERSUS PEDESTRIAN PELVIS
The Hybrid III dummy is usually equipped with a seated pelvis in frontal and side collision testing. Many rollover test programs such as the “Malibu series” ( Bahling et al., 1990 ) utilized a pedestrian pelvis on the dummy because it appeared to offer more realistic dummy kinematics during the rollover. All of the tests presented here included the pedestrian pelvis, with the exception of one seated pelvis dummy that was conducted for comparison. Vertical head excursion for the dummy with the seated pelvis dynamically was slightly less than that for the pedestrian pelvis at lower rotation rates and was identical at the 360 deg/sec rotation rate. A similar comparison of vertical head excursion of Hybrid III dummies with the seated and the pedestrian pelvis was done by Moffatt et al. (1997) using lap belted dummies. They reported slightly more head excursion with the seated than the pedestrian pelvis. It appears that the type of pelvis on a restrained dummy does not have a significant influence on the measured dynamic head excursion.
WEBBING MOVEMENT THROUGH THE LATCH PLATE
During the dynamic tests there was movement of the seatbelt webbing through the latch plate as the subjects moved and changed the relative tensions on the lap and shoulder portions of the belt. Typically this motion resulted in an increase in the length of webbing in the lap belt segment and a decrease in the shoulder belt length. This movement of the webbing toward the lap belt segment was documented photographically and evaluated relative to the head excursion of the subjects. Figure 12 shows data for head excursion versus webbing transfer for static and dynamic near-side tests. Figure 13 shows data for head excursion versus webbing transfer for the corresponding far-side tests. For the near-side roll direction, webbing movement ranged from zero to 50 mm. For the far-side roll direction, webbing movement toward the lap belt segment ranged from 20 to 82 mm.

Near-Side Roll Direction: Head Excursion vs. Webbing Movement through the Latch Plate (Dynamic data at 220 deg/sec)

Far-Side Roll Direction: Head Excursion vs. Webbing Movement through the Latch Plate (Dynamic data at 220 deg/sec).
As the data shows, there is no correlation between head excursion and webbing transfer for either the near or far-side roll directions. While the total length of the two belt segments remained constant, the precise positioning of the latch plate did not affect restraint performance.
SEATBELT LOADS
Maximum lap and shoulder belt loads were measured during the dynamic tests. The highest loads (lap belt = 712 N, shoulder belt = 885 N) occurred with the 50 th percentile dummy at the highest roll rate (360 deg/sec). At the 220 deg/sec rotation rate, the human male subject measured a peak lap belt load of 512 N and shoulder belt load of 463 N. This compared with seatbelt loads for the 50 th percentile dummy of 574 N for the lap belt and 885 N for the shoulder belt under the same conditions.
The position of the shoulder belt webbing relative to the D-ring was monitored photographically. In each test the retractor was allowed to lock normally as the vehicle was being rotated. In all tests the seatbelt remained buckled and the only motion of the webbing at the D-ring was the normal tightening of webbing on the locked retractor. At no time during the dynamic testing did the retractor unlock and allow unspooling of the webbing despite intervals in the vehicle rotation, during the spin-up, where the seatbelt webbing was not loaded by the occupant.
The vehicle rotation fixture used in these tests is a useful tool to measure occupant kinematics in a controlled simulation of the airborne phase of the rollover. It allows the subject to be confined in the space of a complete vehicle, including the production door, seat, steering assembly, instrument panel and seatbelt. The vehicle is rotated about its center of gravity as it would while airborne, and it is rotated at typical roll rates observed in rollover crash tests. However, these tests do not explore the occupant movement that results during vehicle-to-ground impacts in the impact phase of real world rollover collisions. Further, because these tests were conducted with the vehicle supported by a ground-based fixture, the occupant is affected by gravitational forces in a manner different from being in an airborne vehicle. An airborne vehicle and its occupant are both free falling, so there is not a relative force between the occupant and the vehicle as a result of gravity.
While the dynamic dummy testing was conducted at roll rates up to 360 deg/sec, for safety reasons the rotation rate for the human subjects was limited to 220 deg/sec. Because centrifugal force is proportional to the square of the rotational velocity, there is 2.7 times as much centrifugal force at 360 deg/sec than at 220 deg/sec. Additional soft tissue compression and torso extension of the human subjects would be expected at greater rotational velocities.
Finally, the head excursion data presented here represent only the change in vertical position of the head relative to the roofline of the vehicle. Lateral head excursion may influence the likelihood of an occupant having his head partially ejected out the side window leading to ground impact during the rollover.
CONCLUSIONS
- The emergency locking retractor remained locked during rotation, with no unspooling of the webbing. For the human subjects, the 3-point restraint system restricted vertical head movement to about 200 mm dynamically, at 220 deg/sec roll velocity.
- With this typical 3-point restraint system where the shoulder belt fits across the outboard shoulder, testing indicated that occupants positioned on the far-side of a rollover tend to have greater head excursion than occupants positioned on the near-side of a rollover. The outboard shoulder of the far side occupant tended to slip out from beneath the shoulder belt when he leaned inboard away from the shoulder belt.
- For static inversion testing, neither the roll direction, test subject, nor size substantially affected the vertical excursion results. The results of this research show that static inversion test results tend to overestimate near-side dynamic excursion and underestimate far-side dynamic excursion.
- Seatbelt webbing transfer from the shoulder belt to the lap belt through the sliding latch plate was not a predictor of head excursion in these tests.
- The widely varied human subject response was not exhibited with the Hybrid III dummies. Nevertheless, the Hybrid III dummy is a reasonable surrogate for restrained human volunteers under these test conditions when measuring vertical head excursion.
Appendix A. Summary of Data for All Tests
- Arndt MW, Mowry GA, Dickerson CP, Arndt SM. Evaluation of Experimental Restraints in Rollover Conditions. SAE 952712; 1995. [ Google Scholar ]
- Bahling GS, Bundorf RT, Kaspyck GS. Rollover and Drop Tests – The Influence of Roof Strength on Injury Mechanics Using Belted Dummies. SAE 902314; 1990. [ PubMed ] [ Google Scholar ]
- Cooper ER, Moffatt EA, Curzon AM, Smyth BJ, Orlowski KF. Repeatable Dynamic Rollover Test Proceedure with Controlled Roof Impact. SAE 2001-01-0476; 2001. [ Google Scholar ]
- Digges K. Crashworthiness Safety Features in Rollover Crashes. SAE 982296; 1998. [ Google Scholar ]
- Digges KH, Malliaris AC, Ommaya AK, McLean AJ. Characterization of Rollover Casualties. 1991 International Conference on the Biomechanics of Impact; 1991. [ Google Scholar ]
- Friedewald K. An Analysis of Body Loads During Rollover Tests; Roof Crush and Occupant Protection. . 15th ITC on ESV; 1996. [ Google Scholar ]
- Friedman K, Friedman D, Forrest S, Meyer S, Herbst B, Chng D, Wang P. Restraint Effectiveness During Rollover Motion. 1996 IRCOBI Conference; 1996. [ Google Scholar ]
- Hare BM, Lewis LK, Hughes RJ, Ishikawa Y, Iwasaki K, Tsukaguchi K, Doi N. Analysis of Rollover Restraint Performance with and without Seatbelt Pretensioner at Vehicle Trip. SAE 2002-01-0941; 2002. [ Google Scholar ]
- Herbst B, Forrest S, Wang P, Chng D, Friedman D, Friedman K. The Ability of Three-point Safety Belts to Restrain Occupants in Rollover Crashes. 15th ITC on ESV; 1996. [ Google Scholar ]
- Hughes RJ, Lewis LK, Hare BM, Ishikawa Y, Iwasaki K, Tsukaguchi K, Doi N. A Dynamic Test Procedure For Evaluation of Tripped Rollover Crashes. SAE 2002-01-0693; 2002. [ Google Scholar ]
- James MB, Allsop DL, Nordhagen RP, Decker RL. Injury Mechanisms and Field Accident Data Analysis in Rollover Accidents. SAE 970396; 1997. [ Google Scholar ]
- Meyer SE, Davis M, Chng D, Herbst B, Forrest S. Three-Point Restraint System Design Considerations for Reducing Vertical Occupant Excursion in Rollover Environments. SAE 2000-01-0605; 2000. [ Google Scholar ]
- Moffatt EA. Occupant Motion in Rollover Collisions. AAAM 19th Annual Proceedings; 1975. [ Google Scholar ]
- Moffatt EA, Thomas TM, Cooper ER. Safety Belt Buckle Inertial Responses in Laboratory and Crash Tests. SAE 950887; 1995. [ Google Scholar ]
- Moffatt EA, Cooper ER, Croteau JJ, Parenteau C, Toglia A. Head Excursion of Seated Belted Cadever, Volunteers and Hybrid III ATD in a Dynamic/Static Rollover Fixture. SAE 973347; 1997. [ Google Scholar ]
- National Highway Traffic Safety Administration. Traffic Safety Facts 1999. 2000. [ Google Scholar ]
- Orlowski KF, Bundorf RT, Moffatt EA. The Influence of Roof Strength on Injury Mechanics. SAE 851734; 1985. [ Google Scholar ]
- Parenteau C, Gopal M, Viano D. Near and Far-Side Adult Front Passenger Kinematics in a Vehicle Rollover. SAE 2001-01-0176; 2001. [ Google Scholar ]
- Pywell JF, Rouhana SW, McCleary JD, DeSaele KH. Characterization of Belt Restraint Systems in Quasistatic Vehicle Rollover tests. SAE 973334; 1997. [ Google Scholar ]
- Ward CC, Der Avanessian H, Ward P, Paver JG. Investigation of Restraint Function on Male and Female Occupants in Rollover Events. SAE 2001-01-0177; 2001. [ Google Scholar ]

- Maintenance
- Manufacturer
- Personalities
- Business aviation
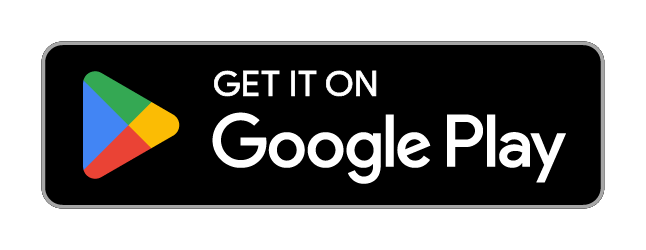
Russian Emergencies Ministry confirms its helicopter’s crash in Moscow region
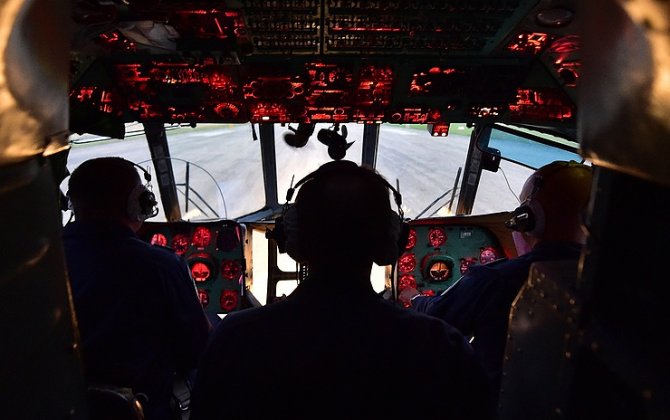
The Russian Emergencies Ministry has confirmed the crash of its helicopter in the Moscow region.
"A Mi-8 helicopter of the Russian Emergencies Ministry crashed during a training flight when the crew was drilling rescue operations in extremely difficult conditions in the Lubertsy district," the Russian Emergencies Ministry press service told TASS.
Three Russian Emergencies Ministry units put on alert
The Russian Emergencies Ministry has put three of its units on alert after the ministry’s Mi-8 helicopter crashed in the Moscow region late on Wednesday.
"The main Emergencies Ministry department for the Moscow region; the ministry’s central regional center and the Zhukovsky-based rescue aviation center have switched over to the emergency situation regime in the connection with the Mi-8 crash," the the ministry’s central regional center told TASS.
A regional law enforcement source told TASS two pilots and a flight engineer were onboard.
"The crew’s captain, Roman Frolov, the head of the Zhukovsky-based Emergencies Ministry rescue aviation center; his deputy pilot Dmitry Arsentyev and flight engineer Maxim Filippov," the source said adding that Frolov had been Arsentyev’s instructor.
"They drilled rescue and monitoring operations in difficult weather conditions at night time," the source stressed.
The crew tried to take the vehicle away from highways and populated localities
The crew of the Russian Emergencies Ministry Mi-8 helicopter, which crashed in the Moscow region late on Wednesday could have tried to take the vehicle away from highways and populated localities when they understood that a technical breakdown might have occurred onboard, an aviation source told TASS.
"It looks as if the crew tried to lead the helicopter away from the populated localities. It fell down in the Tomilino forest park between the settlements of Mirnyi and Oktyabrsky - just one kilometer away from Oktyabrsky and 1.5 kilometers away from the Lytkarino highway," the source explained.
According to preliminary reports, there are no casualties or destruction on the ground. The crashed Mi-8 helicopter had been stationed at an air base in Zhukovsky near Moscow, which is a home to the Russian Emergencies Ministry aviation unit.
The accident occurred in the Lubertsy district of the Moscow region on Wednesday night. The fire at the crash site has been extinguished. The bodies of three crew members have been found. The Russian Investigation Committee has started a pre-trail investigation.
Recommended
Pearl 10x engine took to the skies for the first time.
Rolls-Royce has successfully kicked off the flight test campaign for its latest aero engine for the business aviation market, the Pearl 10X, on the company’s dedicated Boeing 747 flying tes...
Sonoma Jet Center champions sustainable aviation with Green Fuel Day Event
In an ambitious stride toward sustainable aviation, Sonoma Jet Center at Charles M. Schulz – Sonoma County Airport (KSTS), announces its upcoming Green Fuel Day Celebration on April 18, 202...
TCab Tech closes Series A financing with additional 20 million USD
TCab Tech announces it has raised an additional 20 million USD in its Series A financing. The exclusive investment comes from a strategic investment fund which plans to introduce the air-tax...
Inbound to Horn Island, Australia first P2012 Travellers
Torres Strait Air, based on Horn Island, in Far North Queensland, is the launch customer for the twin engine, 11-seat, Tecnam P2012 Traveller, in Australia. TSA will take delivery of two P2012s i...
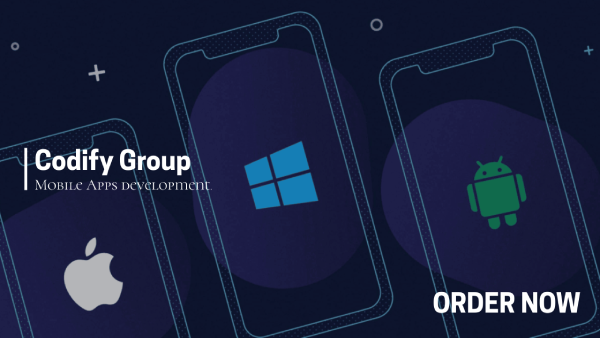
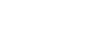
- Yekaterinburg
- Novosibirsk
- Vladivostok
- Tours to Russia
- Practicalities
- Russia in Lists
Rusmania • Deep into Russia
Moscow metro excursion
Plan your next trip to russia, ready-to-book tours.
Your holiday in Russia starts here. Choose and book your tour to Russia.
REQUEST A CUSTOMISED TRIP
Looking for something unique? Create the trip of your dreams with the help of our experts.

IMAGES
VIDEO
COMMENTS
Excursions so dominate the group of very large 4-wheel-drive SUVs that they push up this group's average death rate to 76 per million compared with 47 in large counterpart vehicles and 59 in midsize ones. About half of the deaths in 2001-04 model Excursions during 2002-05 occurred in rollover crashes." Reply Like.
The Side Barrier test simulates an intersection collision between a standing vehicle and moving barrier at 38.5 mph. The Side Pole Barrier test simulates a crash into a fixed object like a tree or utility pole. The Rollover Resistance test measures the risk of rollover in a single-vehicle, loss-of-control scenario.
Cruising along in a 2004 Ford Excursion gives you a secure feeling. The optional V10 ($585) is a delightful engine. It produces 310 horsepower and generates a very impressive 425 pounds-feet of ...
Vehicle ratings. IIHS tests evaluate two aspects of safety: crashworthiness — how well a vehicle protects its occupants in a crash — and crash avoidance and mitigation — technology that can prevent a crash or lessen its severity. Start typing a make and/or model, and a list of choices will appear. You can specify a year too.
Excursion - King of SUVs - Excursion crash ability - I've seen it mentioned a few times about how these are so great in collisions due to their size and bulk but I have not been able to find any collision related safety reports or testing on these trucks. ... All the full sizes were fairly comparable in rollover tests, the drop test is a dumb ...
These ratings are developed by NHTSA and are made to give you confidence and security when you hit the road. The more stars your Ford Excursion have, the safer it would be! If you want to check its safety features for crash prevention, select the year of manufacture. We arranged our charts with everything from door curb lights to stolen vehicle ...
MSRP. $49,690. Engine. 6.0L V-8. MPG. city / hwy. Explore Build & Price. Review the 2003 Excursion Limited 6.0L 4x4 crash safety ratings from the IIHS and NHTSA to see how well passengers are ...
The IIHS has not conducted crash tests for the 2002 Excursion yet. Ratings only apply to the model and body or seat type tested. The date of manufacture and optional safety equipment can affect ...
Eddie Bauer Sport Utility 4D. $44,985. $13,298. Limited Sport Utility 4D. $46,050. $14,514. For reference, the 2004 Ford Excursion originally had a starting sticker price of $40,485, with the ...
Impact Speed and Overlap. Small overlap barrier crash tests are conducted at 64.4 ± 1 km/h (40 ± 0.6 mi/h) and 25 ± 1% overlap. The test vehicle is aligned with the rigid barrier such that the right edge of the barrier face is offset to the left of the vehicle centerline by 25 ± 1% of the vehicle width (Figure 1).
Eddie Bauer Sport Utility 4D. $45,315. $18,037. Limited Sport Utility 4D. $46,640. $14,979. For reference, the 2005 Ford Excursion originally had a starting sticker price of $41,395, with the ...
When people think of crash test dummies, they often associate it with: "You Could Learn a Lot From a Dummy.". This famous tagline was the foundation of a popular NHTSA safety campaign urging people to wear their seat belts. Vince and Larry, the loveable crash test dummies, encouraged seat belt use through TV, print, and radio ads.
Excursion - King of SUVs - Truth about crash test ratings - This has been bugging me for a while and I finally found out the answer to how and why the S(tupid)mart For Two could possibly get a 5 star front crash test rating. ... Excursion - King of SUVs 2000 - 2005 Ford Excursion. Sponsored by: 11-19-2014, 02:28 PM How-Tos on this Topic Last ...
To compare the human and crash test dummy response, the research presented here measured the static and dynamic vertical head excursion of four human subjects and similar sized dummies in simulated airborne rollover conditions, including both far-side and near-side roll directions. ... The head excursion was determined from post-test review of ...
This video shows the weak roof structure of a 2000 Ford Excursion. Nearly 220,000 rollover accidents occur in the U.S. every year, many of which cause seriou...
Moscow authorities have closed the western Slavyansky Bulvar and Park Pobedy metro stations on the Dark Blue Line and restricted traffic on the thoroughfare Kutuzovsky Prospekt until at least 10 p.m.
At Least 21 Killed in Moscow Metro Rush-Hour Crash. July 15, 2014 Members of the emergency services carry an injured passenger outside a metro station following an accident on the subway in Moscow
The Russian Emergencies Ministry has confirmed the crash of its helicopter in the Moscow region. "A Mi-8 helicopter of the Russian Emergencies Ministry crashed during a training flight when the crew was drilling rescue operations in extremely difficult conditions in the Lubertsy district," the Russian Emergencies Ministry press service told TASS.
Choose district. and we'll show you all the information you need