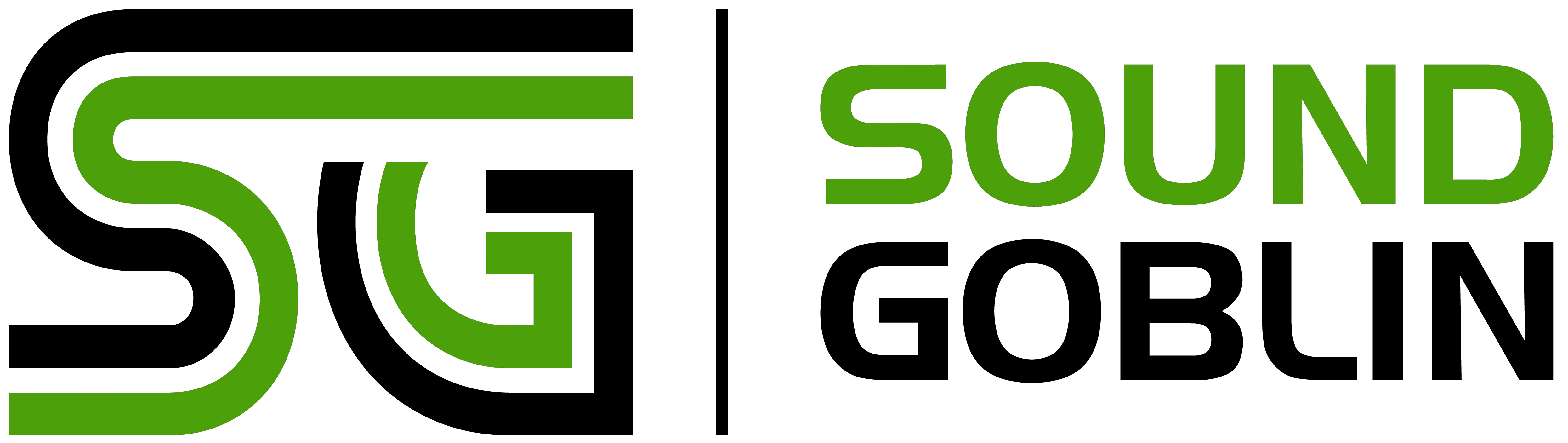

How Sound Travels Through Solids, Liquids and Gases
- August 13, 2022
We love to work with sound. Many of us record our own music, podcast, or other forms of sound. Knowing how sound travels through different mediums will allow you to have better control over the sound that you generate. That is what we will be looking at today. How does sound travel through solids, liquids, and gases?
Can the way that you produce sound and the medium that it moves in make a difference in the volume that you will hear? How does this change when it comes to the different mediums? Will the furniture in a room have any impact on the acoustics of the room? How can you change it to create the perfect recording environment?
These are just some of the things that we will discuss today. Knowing how sound travels through solids, liquids and gases are not only interesting, but it can have an impact on the way we record sounds and how we change things up.
Why Is the Way That Sound Travels Through Mediums Important?
One of the main reasons why it is important to understand how sound acts, is that when you understand something better, you can control it. As a youngster, I loved swimming. I still do. But one of my main attractions was that underneath the water it was the one place where everything went quiet. It always felt like the world stopped and it was just me and complete calm.
I loved my family but there was always so much going on that it was just a great place to just be with my own imagination and thoughts. I could make up imaginary worlds and people, and have millions of stories running through my mind. All because there was a lack of sound under the water.
But in reality, when you look at how sound behaves in a liquid, scientifically, this should not be the case. In fact, there should be more sound under the water than there is in the fresh air. Why? And why doesn’t it work that way? I wanted to find out.
For many of us who record sound, it is important to be connected to it. If you understand what makes something sound fuller, what makes a noise loud, and how things act, you can have better control and your recording will end up being closer to what you intended in the first place.
You might be recording a podcast, but for some reason, your voice keeps sounding muffled, understanding sound can help you identify what the cause could be and how you can fix it.
What Is Sound?
To understand why sound acts differently in different mediums we first have to understand what sound is.
First, you need to know that sound can not exist in a void. This is different from light that does travel through nothingness. That is why we can see light shining from space where there is a void and no atmosphere. But those that have been to space say it is completely quiet. It must be an almost eerie feeling.
Sound happens when something creates a vibration. This is done through musical instruments, our voices, speakers, and many other things. This then causes the medium around it, like the water or the air, to also vibrate and carry this sound with them. Without a medium, sound would not exist. That is because the molecules of the medium react and bump into those next to it and this allows the sound to travel on.
At the same time, the medium that is used will determine just how loud the sound will be, it will also determine how far can travel and how the sound will generally react. This is because different solutions will have molecules that are more or less densely packed.
Your surroundings will have a big impact. People who create a sound studio try to make the acoustics of the room as powerful as possible. This should help you do that.
Let us now look at the three mediums that sound can travel through, solids, liquids, and gases, and how they change the reaction of the sound.
First up we can look at gasses. You might wonder why gasses are mentioned when speaking of mediums that sound can move in. You may be visualizing a bunch of fog at a concert that makes the lights look incredible and makes the crowd go wild.
And that is one possibility of gasses that can be used as a medium for sound to travel through. But most of the time, our air is the only gas that sound needs to continue its vibration.
What Is the Air Made Of?
We have already mentioned that sound can not exist in a void. But we can hear each other when we speak out in the open. We can hear music when it is being played under the starry sky and we can even hear kids shouting in a park a block away .
That is because most of the air in our atmosphere is made up of gasses. Our atmosphere is not just a void, or we wouldn’t be able to live here anyway, but is made up of lots of gasses we can’t see. The atmosphere is made up of 78% Nitrogen, and 21% Oxygen, and the rest is a mixture of carbon dioxide, neon, and hydrogen.
This gives us all the ability to breathe without needing a space suit, but it also gives sound the ability to travel in our atmosphere. We make a vibration and the molecules of the gases that we can’t even see start to bump into each other and takes that vibration further, making it possible for us to hear sounds. It is pretty amazing when you think about it.
How Does Sound Travel Through Gases?
Gas is the medium that will have the slowest speed of sound of all of them. This is because the molecules of the gases surrounding us are expanded and far away from each other. The vibrations do get passed over to each other but it takes longer to do.
This is also why we often need things that can amplify our sounds like a microphone when we are speaking to a bigger group of people. These help us to make the vibrations bigger and to allow them to travel further than we would have been able to achieve with only our voice.
Some Things That Can Influence Sound in Gases
Have you ever felt that things are so much quieter after a big snowstorm? How the world seems almost different then? Turns out it might not just be your imagination. This is because the volume and speed of sound can be impacted by the temperature of the air and in turn the gas that is surrounding us.
At lower temperatures, the molecules move around quicker and they can vibrate quicker. The energy behind the sound can start to be lost and the sound will become quieter or be lost faster.
At normal room temperatures, the speed of sound will be a lot higher than it would be in the exact same room when the temperature is at freezing.
There are many different liquids that have a higher or lower density but for the most part, it is in water where we would be interested in hearing a sound. If we go swimming or put a small portable speaker close to the water, we would like to hear the sound as loud as possible. But it just doesn’t always work like that.
Let’s see how sound reacts in water or other liquids.
Sound In Water
The molecules in water are a lot more tightly packed than it is in gas. That is why sound travels much faster in water than it would travel in the air. Sound can actually travel in water almost four times faster than it can be in the air.
That is really impressive. And still, if you submerge your head underwater, you will hear the sound but it might sound muffled and not quite like the sound that you are used to.
Why Humans Hear Muffled Sounds in Water
The water molecules are more tightly packed and the energy that it uses to carry sound is transported faster. In theory, you should be able to hear noises a lot louder when you are underwater. But that is not how we perceive this sound.
This is because our ears are created to listen to sounds in the air. We pick up on sounds through our ear canal and these sounds are then transported to the brain that makes sense of it all. When you only submerge your ears, sounds will sound very muffled since the ears can’t take these sounds along the ear canal.
When you submerge your head fully suddenly the sound is clearer and louder. Although it could still be somewhat muffled compared to outside the water. Our heads contain a lot of water, and inside the water, it will be our tissue that picks up on the sound and detects it.
You could try to plug your ears but it will have very little effect on the volume of the noise under the water. The sound is not traveling along those normal lines.
At the same time, chances are that it is also very hard and almost impossible for you to figure out from which direction the sound is coming. When the sound travels along the normal route our brain has cues to determine if it comes from behind us or in front. But when the sound does not travel in those normal routes the brain has no way of telling us where it is coming from.
For humans communicating through sound under the water is not so easy. That is why divers have always used hand signals to communicate with their diving partners and why some have even started to use microphones that connect them. Allowing for a much better communication route.
We know that we can’t hear sound in the same way when we are inside water as when we are in the air. But what happens when we make a big sound inside the water, like shouting? Will someone that is on the outside be able to hear it clearly?
This is unlikely. That is because the surface of the water almost acts as a mirror for sound. Instead of the vibration moving outside of the water it gets reflected back. Making sure that very little sound is heard outside.
Animals In Water
Our ears might be designed to hear in air, but fish and mammals that live in the ocean can take advantage of the speed of sound inside the water. They are adapted to hearing noise completely clearly inside the water.
Since sound does travel quickly in water and they can hear it, they can use sounds to communicate over much larger distances than we are able to do with just our voice. Whales, for example, have been known to use their voice to communicate with other whales over massive distances in the ocean. The sound of a humpback whale can travel thousands of miles in the ocean. It also helps that the vibrations they can create are much larger than the ones our own vocal cords can produce.
Then finally there are solids and how sound reacts when they come into contact with a solid. Since sound starts to get muffled when there are a lot of solid objects in its path you would think that it travels a lot slower in solids. But surprisingly that is not the case. There are however reasons why it reacts in this way.
The Speed of Sound in Solids
A solid object is densely packed with its molecules. Each solid object will be a little bit different from the other depending on the material it is made of and how densely packed its molecules are. There are some materials that will work better as insolation to noise than others, but we will discuss the reason for this shortly. But for the most part, sound will travel a lot faster in solids than it will in both liquids and gasses.
This is because the source of the sound will create the vibration in the molecules of the sound and then these tightly packed molecules will quickly send the vibration further along. This means that the speed of sound is a lot faster when traveling in a solid object and that it will be a lot louder too.
Often a solid object will be a good source of amplification for a sound that you would like to enhance. The sound through a brass bugle gets enhanced through the design of the object and also through the material it is made of.
Examples Of Sounds in Solids
It can be hard to think of examples where solid objects are used to move sound and make it louder. Let’s discuss some simple examples of this.
You can put an ear to a solid object like a table and then make a soft tapping sound on the table. Compare how you heard it when your ear is on the solid compared to how loud it is when you hear the sound through the air. You will be surprised by how clearly the sound is enhanced by listening to it through a solid object.
Another great example of an experiment that many of us probably unknowingly did as children is a string telephone. You take two cups and a long line of string. The two cups are each connected to one side of the string, one person listens into one cup while another speaks into the cup at their end.
In this experiment, the vibrations are created and enhanced by the shape of the cup. Then these vibrations are transferred with the help of a solid object, the string, and the other person can hear your message at the other end of the string. Without raising your voice or shouting.
It is always amazing to see just how far this simple design can carry sound. Fun fact, the world record for the longest-ever string telephone, which was made with tin cans, was a whopping 796 feet long. That is almost the distance of three football fields. That is a long way for a piece of string and two cups to carry sound.
Then another great example of a sound being a lot louder when it is carried through a solid object is sounds that you can hear in the air. For example, hearing the sound of a horse coming closer, its hooves beating down on the ground.
It is already a pretty impressive sound when you hear it in normal circumstances. But try putting your head to the ground and listening to the approach in that way. The sound is much louder and you can almost feel the vibrations that are making the sound you hear.
Why Does Sound Get Muffled Through a Door?
We know now that sound travels much faster through solid objects than it does through gasses or liquids. You would think that a solid object like a wall or a door will enhance the sound but the opposite is true. A sound that is coming from a different room is more muffled.
If there is a lot of noise outside your home, for example, the neighbors having a party, it works to close the doors and windows and the sound won’t bother you as much. Even if you only have standard windows and doors.
How does that work? It works because the sound you are hearing does not originate from inside a solid object. It traveled through the air until it came to your door. There it encountered a solid object. And instead of making the vibrations louder this change in medium made the sound lose some of the energy that it was traveling with. This reduces the level of the noise and makes it less noticeable when there are doors that are closed.
Why Rooms Echo
This change in energy is also one of the reasons why a room will or won’t echo. When you go into an empty room there is a good chance that you can create an echo. That is because the empty room has no solid objects that break the energy of the noise down and stop it.
The vibrations bump only against the walls and reflect back. If you have a room that is still echoing even after your furniture has been installed, then there might not be enough solid objects that stop the speed and the energy of the sound. Something like a carpet that can absorb the vibration can help to stop the echo in the room.
How Sound Travels Through Solids, Liquids, and Gases
Sound needs a medium that can take the vibrations and move them along, allowing us to hear the sounds that are being created.
When it comes to the speed of sound, a solid object will allow the vibration to move much faster since it has the most densely packed molecules. It will also make the sound the loudest. After solids, liquids have the highest speed of sound. And then finally gas, that included our air since it is made up of gasses.
When a sound is traveling through one medium like air and then encounters another, like a solid door, it loses some of its energy and some of the volume will be lost. That is why solid insulation against sound is still one of the best options despite solids being a good conductor of sound.
We might not be able to take full advantage of the high speed of sound that can be found inside a liquid, but those living in the ocean sure can and that is why whale sounds can travel thousands of miles under the water.
Knowing how sound reacts to different mediums will allow us to understand it better. And that means that you should have better control over your recordings and all the ways that you like to create your own very unique sounds.
Leave a Reply Cancel Reply
Add Comment
Save my name, email, and website in this browser for the next time I comment.
Post Comment
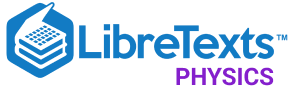
- school Campus Bookshelves
- menu_book Bookshelves
- perm_media Learning Objects
- login Login
- how_to_reg Request Instructor Account
- hub Instructor Commons
- Download Page (PDF)
- Download Full Book (PDF)
- Periodic Table
- Physics Constants
- Scientific Calculator
- Reference & Cite
- Tools expand_more
- Readability
selected template will load here
This action is not available.
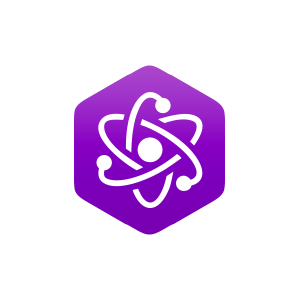
- You do not have permission to view this page - please try signing in.
- Password reset
Welcome to the LibreTexts library. For other users, please log in with LibreOne.
- Sound Waves
- Speed Of Sound Propagation
Speed of Sound
A sound wave is fundamentally a pressure disturbance that propagates through a medium by particle interaction. In other words, sound waves move through a physical medium by alternately contracting and expanding the section of the medium in which it propagates. The rate at which the sound waves propagate through the medium is known as the speed of sound. In this article, you will discover the definition and factors affecting the speed of sound.
Speed of Sound Definition
The speed of sound is defined as the distance through which a sound wave’s point, such as a compression or a rarefaction, travels per unit of time. The speed of sound remains the same for all frequencies in a given medium under the same physical conditions.
Speed of Sound Formula
Since the speed of sound is the distance travelled by the sound wave in a given time, the speed of sound can be determined by the following formula:
v = λ f
Where v is the velocity, λ is the wavelength of the sound wave, and f is the frequency.
The relationship between the speed of sound, its frequency, and wavelength is the same as for all waves. The wavelength of a sound is the distance between adjacent compressions or rarefactions . The frequency is the same as the source’s and is the number of waves that pass a point per unit time.
Solved Example:
How long does it take for a sound wave of frequency 2 kHz and a wavelength of 35 cm to travel a distance of 1.5 km?
We know that the speed of sound is given by the formula:
v = λ ν
Substituting the values in the equation, we get
v = 0.35 m × 2000 Hz = 700 m/s
The time taken by the sound wave to travel a distance of 1.5 km can be calculated as follows:
Time = Distance Travelled/ Velocity
Time = 1500 m/ 700 m/s = 2.1 s
Factors Affecting the Speed of Sound
Density and temperature of the medium in which the sound wave travels affect the speed of sound.
Density of the Medium
When the medium is dense, the molecules in the medium are closely packed, which means that the sound travels faster. Therefore, the speed of sound increases as the density of the medium increases.
Temperature of the Medium
The speed of sound is directly proportional to the temperature. Therefore, as the temperature increases, the speed of sound increases.
Speed of Sound in Different Media
The speed of the sound depends on the density and the elasticity of the medium through which it travels. In general, sound travels faster in liquids than in gases and quicker in solids than in liquids. The greater the elasticity and the lower the density, the faster sound travels in a medium.
Speed of Sound in Solid
Sound is nothing more than a disturbance propagated by the collisions between the particles, one molecule hitting the next and so forth. Solids are significantly denser than liquids or gases, and this means that the molecules are closer to each other in solids than in liquids and liquids than in gases. This closeness due to density means that they can collide very quickly. Effectively it takes less time for a molecule of a solid to bump into its neighbouring molecule. Due to this advantage, the velocity of sound in a solid is faster than in a gas.
The speed of sound in solid is 6000 metres per second, while the speed of sound in steel is equal to 5100 metres per second. Another interesting fact about the speed of sound is that sound travels 35 times faster in diamonds than in the air.
Speed of Sound in Liquid
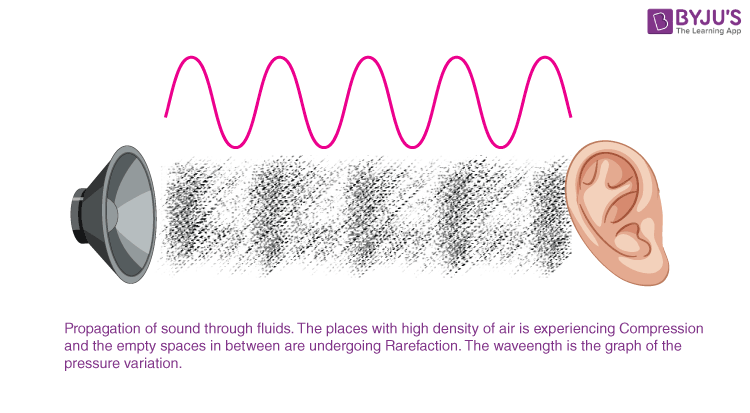
Speed of Sound in Water
The speed of sound in water is more than that of the air, and sound travels faster in water than in the air. The speed of sound in water is 1480 metres per second. It is also interesting that the speed may vary between 1450 to 1498 metres per second in distilled water. In contrast, seawater’s speed is 1531 metres per second when the temperature is between 20 o C to 25 o C.
Speed of Sound in Gas
We should remember that the speed of sound is independent of the density of the medium when it enters a liquid or solid. Since gases expand to fill the given space, density is relatively uniform irrespective of gas type, which isn’t the case with solids and liquids. The velocity of sound in gases is proportional to the square root of the absolute temperature (measured in Kelvin). Still, it is independent of the frequency of the sound wave or the pressure and the density of the medium. But none of the gases we find in real life is ideal gases , and this causes the properties to change slightly. The velocity of sound in air at 20 o C is 343.2 m/s which translates to 1,236 km/h.
Speed of Sound in Vacuum
The speed of sound in a vacuum is zero metres per second, as there are no particles present in the vacuum. The sound waves travel in a medium when there are particles for the propagation of these sound waves. Since the vacuum is an empty space, there is no propagation of sound waves.
Table of Speed of Sound in Various Mediums
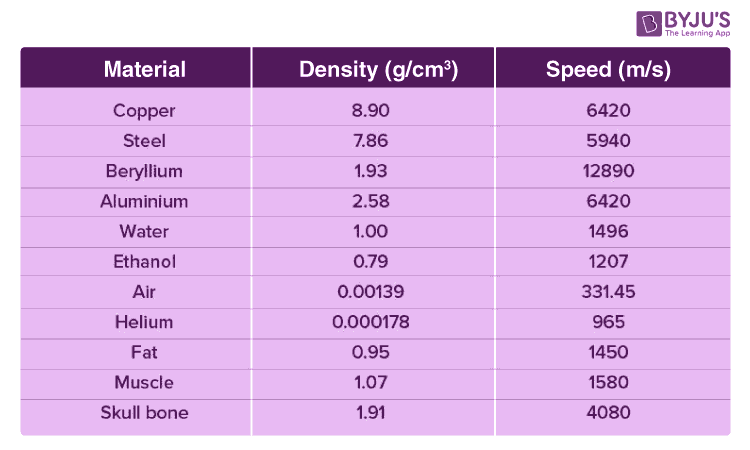
Another very curious fact is that in solids, sound waves can be created either by compression or by tearing of the solid, also known as Shearing. Such waves exhibit different properties from each other and also travel at different speeds. This effect is seen clearly in Earthquakes. Earthquakes are created due to the movement of the earth’s plates, which then send these disturbances in the form of waves similar to sound waves through the earth and to the surface, causing an Earthquake. Typically compression waves travel faster than tearing waves, so Earthquakes always start with an up-and-down motion, followed after some time by a side-to-side motion. In seismic terms, the compression waves are called P-waves, and the tearing waves are called S-waves . They are the more destructive of the two, causing most of the damage in an earthquake.
Visualise sound waves like never before with the help of animations provided in the video
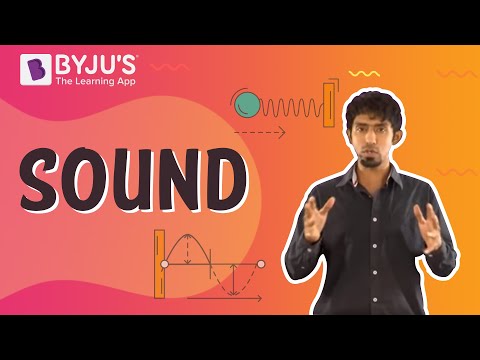
Frequently Asked Questions – FAQs
What is the speed of sound in vacuum, name the property used for distinguishing a sharp sound from a dull sound., define the intensity of sound., how does the speed of sound depend on the elasticity of the medium, why is the speed of sound maximum in solids, name the factors on which the speed of sound in a gas depends., what is a sonic boom, the below video helps to completely revise the chapter sound class 9.
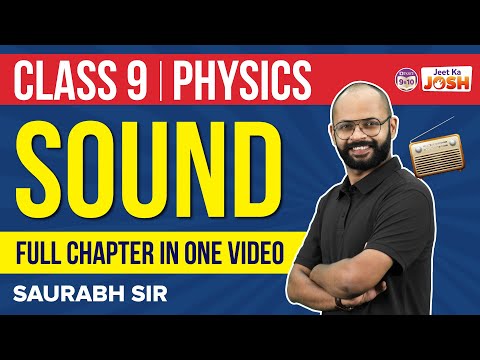
Stay tuned to BYJU’S and Fall in Love with Learning !
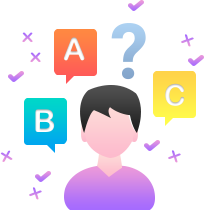
Put your understanding of this concept to test by answering a few MCQs. Click ‘Start Quiz’ to begin!
Select the correct answer and click on the “Finish” button Check your score and answers at the end of the quiz
Visit BYJU’S for all Physics related queries and study materials
Your result is as below
Request OTP on Voice Call
Leave a Comment Cancel reply
Your Mobile number and Email id will not be published. Required fields are marked *
Post My Comment

- Share Share
Register with BYJU'S & Download Free PDFs
Register with byju's & watch live videos.

FREE K-12 standards-aligned STEM
curriculum for educators everywhere!
Find more at TeachEngineering.org .
- TeachEngineering
- Traveling Sound
Hands-on Activity Traveling Sound
Grade Level: 4 (3-5)
Time Required: 30 minutes
Expendable Cost/Group: US $2.00
Group Size: 2
Activity Dependency: None
Subject Areas: Physical Science, Reasoning and Proof, Science and Technology
NGSS Performance Expectations:
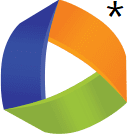
Curriculum in this Unit Units serve as guides to a particular content or subject area. Nested under units are lessons (in purple) and hands-on activities (in blue). Note that not all lessons and activities will exist under a unit, and instead may exist as "standalone" curriculum.
- Seeing and Feeling Sound Vibrations
- Pitch and Frequency
- Sound Visualization Stations
TE Newsletter
Engineering connection, learning objectives, materials list, worksheets and attachments, more curriculum like this, pre-req knowledge, introduction/motivation, vocabulary/definitions, troubleshooting tips, activity extensions, activity scaling, user comments & tips.
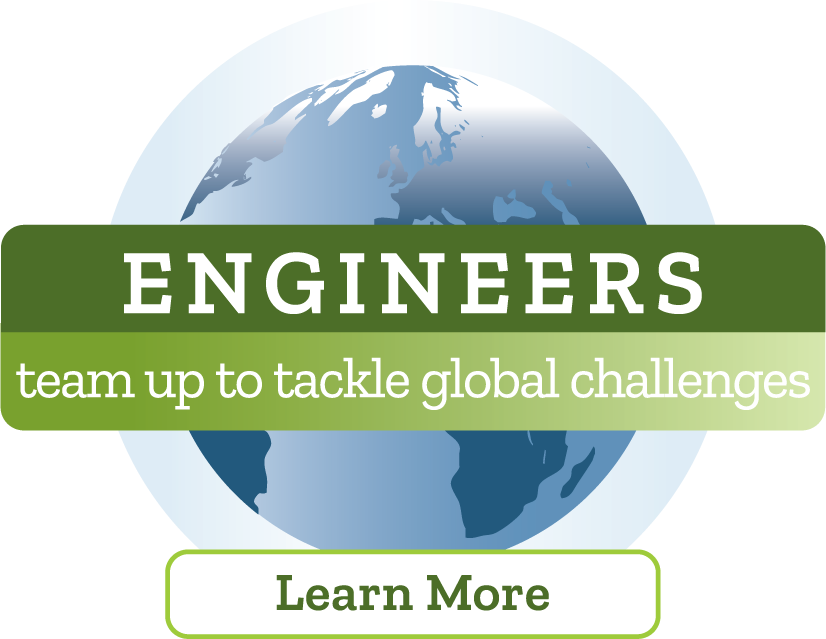
Sound and acoustic engineers know that the shape of a room and its materials greatly impact how sound waves travel. Recording studios are designed in soundproof booths so that the recorded music does not contain any unwanted external noise. Libraries are designed to reduce any introduced noises, to assure a quiet, non-distracting learning environment. Concert halls are designed so that sound generated on the stage travels to the back of the space without being distorted.
After this activity, students should be able to:
- Explain that sound can move through solids, liquids and gases.
- Describe how sound needs molecules to move and that changing the medium that it travels through changes the sound.
- Describe how engineers use sound energy when designing spaces, such as movie theaters.
Educational Standards Each TeachEngineering lesson or activity is correlated to one or more K-12 science, technology, engineering or math (STEM) educational standards. All 100,000+ K-12 STEM standards covered in TeachEngineering are collected, maintained and packaged by the Achievement Standards Network (ASN) , a project of D2L (www.achievementstandards.org). In the ASN, standards are hierarchically structured: first by source; e.g. , by state; within source by type; e.g. , science or mathematics; within type by subtype, then by grade, etc .
Ngss: next generation science standards - science, international technology and engineering educators association - technology.
View aligned curriculum
Do you agree with this alignment? Thanks for your feedback!
State Standards
Colorado - science.
Each group needs:
- large bowl (metal works best)
- 2 metal objects, such as spoons, to knock together
- Traveling Sound Worksheet , one per student
A basic understanding of the phases of matter: liquids, solids and gases.
Sound engineers are especially interested in the way sound travels. Can you hear as well when you sit in the back of the class as when you sit in the front? What about in the assembly hall or gymnasium? On the playground? Can you think of other times when you cannot hear as well as someone else? What happened? How about in a movie theater? What do engineers do so that the sound quality is good for everyone in a movie theater? (Possible answers: Add speakers around the room, curtains, carpet the walls, cone-shaped theaters act like a megaphone and help to direct sound waves further.)
Which is louder—walking on carpet or on tile? It is quieter on carpet because the carpet absorbs the sound energy . Sound energy, light energy and other types of energy, need molecules to travel through and vibrate , but sometimes sound energy is absorbed by an object or material. Engineers use this idea when designing rooms that are meant to be quiet. Have you ever noticed how the walls of a movie theater are covered with carpet or fabric? This is to prevent echoing of the sound system. Sometimes when you are in an empty room, your voice echoes or sounds hollow. This is because an empty room has no materials in it that might absorb the sound energy, so the sound bounces off the hard walls, back at you. This makes it hard to hear clearly.
Do you think sound energy can travel through air? Of course it can! That is how sound energy travels when you talk to a friend. How about water? Can you hear sound travel under water? How about a solid? Can sound move through a solid object? Engineers want to know if sound can travel through solids, liquids and gases so they can develop ways to send messages to people all over the world. Can you imagine how great sound would be if it could travel anywhere?
Understanding the properties of sound and how sound waves travel helps engineers determine the best room shape and construction materials when designing libraries, classrooms, sound recording studios, concert halls and theatres. Room shape and materials can impact how sound waves travel since sound waves bounce off different object in different ways. In this activity, we are going to study how sound waves travel through liquids, solids and gases, and think about how engineers might use this information.
Before the Activity
- Gather materials and make copies of the Traveling Sound Worksheet .
- Divide the class into teams of two students each.
With the Students
- Ask the students to predict if sound can move through solids, liquids and gases.
- Have the students complete the worksheet, which leads them through traveling sound wave activities.
- Can sound energy travel through solids? Students place their ears on a desk or table as they tap or scratch on the top. They compare that to the same sound made when their ear is not pressed to the table.
- Can sound energy traveling through liquids? Fill a large bowl or bucket (metal works best) with water. One student taps two spoons together under the water. Two other students observe and compare the tapping sound they hear, as heard through the air and as heard by placing an ear against the bowl.
- Can sound energy traveling through gases (air)? The students feel their throats gently during each of these tasks:
- Hum with your mouth and nose open.
- Hum with your mouth open and nose closed.
- Hum with your mouth closed and nose open.
- Hum with your mouth and nose closed.
- Discuss with the students what happened. Were their predictions correct? Can sound travel through air, water and solids? (Answer: Yes!) Sound needs molecules to move. Solids, liquids and gases are all made of molecules. The characteristics of the molecules (for example, the space between the molecules) determine whether the sound becomes muffled or changes in some way.
- How might engineers use the knowledge that sound travels through solids, liquids and gases? (Possible answers: Engineers create devices that send sound anywhere — through water to a submarine in the ocean, through wires to your TV, and through the air in surround sound movie theaters or emergency broadcast signals.)
echo: Repetition of a sound by reflection of sound waves from a surface.
frequency: The rate of vibrations in different pitches.
pitch: The highness or lowness of a sound.
sound energy: Audible energy that is released when you talk, play musical instruments or slam a door.
sound wave: A longitudinal pressure wave of audible or inaudible sound.
vibration: When something moves back and forth, it is said to vibrate. Sound is made by vibrations that are usually too fast to see.
volume: When sound becomes louder or softer.
wave: A disturbance that travels through a medium, such as air or water.
Pre-Activity Assessment
Prediction: Ask students if they think sound can move through solid, liquid, and gas. If so what are some examples? (Possible examples: Students may recall talking under water or using tin can and string telephones.)
Activity Embedded Assessment
Worksheet: Have students use the Traveling Sounds Worksheet to guide them in the activity and as a place to record their observations. Review their answers to gauge their mastery of the subject.
Post-Activity Assessment
Toss-a-Question: Ask students to independently think of an answer to the question below and write it on a half sheet of paper. Have students wad up and toss the paper to another team member who then adds their answer idea. After all students have written down ideas, have them toss the paper wad to another team, who reads the answers aloud to the class. Discuss answers with the class.
- What is an example of something through which sound can travel?
Neighbor Check: Have the students compare their activity observations with a neighbor. Are they the same or different? Have each team report some of their similar and dissimilar observations to the rest of the class.
Engineering Design: The supply of air on Earth is running out! Several futuristic cities for human habitation are being designed either underwater or deep inside mountains. Have each student group become a city planning engineering team and draw a communication system for sending emergency messages between the new cities. Make sure to illustrate and describe how the sound energy (message) will move through air, water or solid rock.
This activity can be very loud. Ask students to not disturb others while they learn and have fun.
To bring some humor to the activity, ask each student to hum a small part of their favorite song while feeling their throat. Have each student alternate between having their nose and mouth open or closed while humming non-stop. Why does the sound change depending on whether you close your nose or mouth? What happens if you block your ears? What does this activity teach us about sound? (Answer: Sound vibrations must travel through air for us to hear them. Like a musical instrument [perhaps a recorder or flute], if you change the holes where sound escapes, it changes the pitch, but not the frequency/vibrations of the sound.)
If a metal bowl is used during the activity, the vibrations from the objects colliding underwater vibrate the bowl, creating the illusion that the bowl is being struck. Have students draw the vibrations in the bowl on a piece of paper. Do the vibrations change if the objects are tapped together increasing softly?
Have students think about different forms of communications. Does sound travel most often through solids, liquids or gases? Have students poll their friends, family and neighbors to solicit their ideas.
For lower grades, conduct the activities as a class instead of in teams. Younger students could also draw pictures of their observations instead of writing in sentence form.

Students are introduced to the sound environment as an important aspect of a room or building. Several examples of acoustical engineering design for varied environments are presented.

Students learn how different materials reflect and absorb sound.

Students learn that sound is energy and has the ability to do work. Students discover that sound is produced by a vibration and they observe soundwaves and how they travel through mediums. They understand that sound can be absorbed, reflected or transmitted.
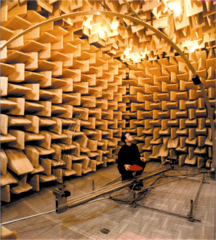
Students use the engineering design process to design and create soundproof rooms that use only one type of material. They learn and explore about how these different materials react to sound by absorbing or reflecting sound and then test their theories using a box as a proxy for a soundproof room. ...
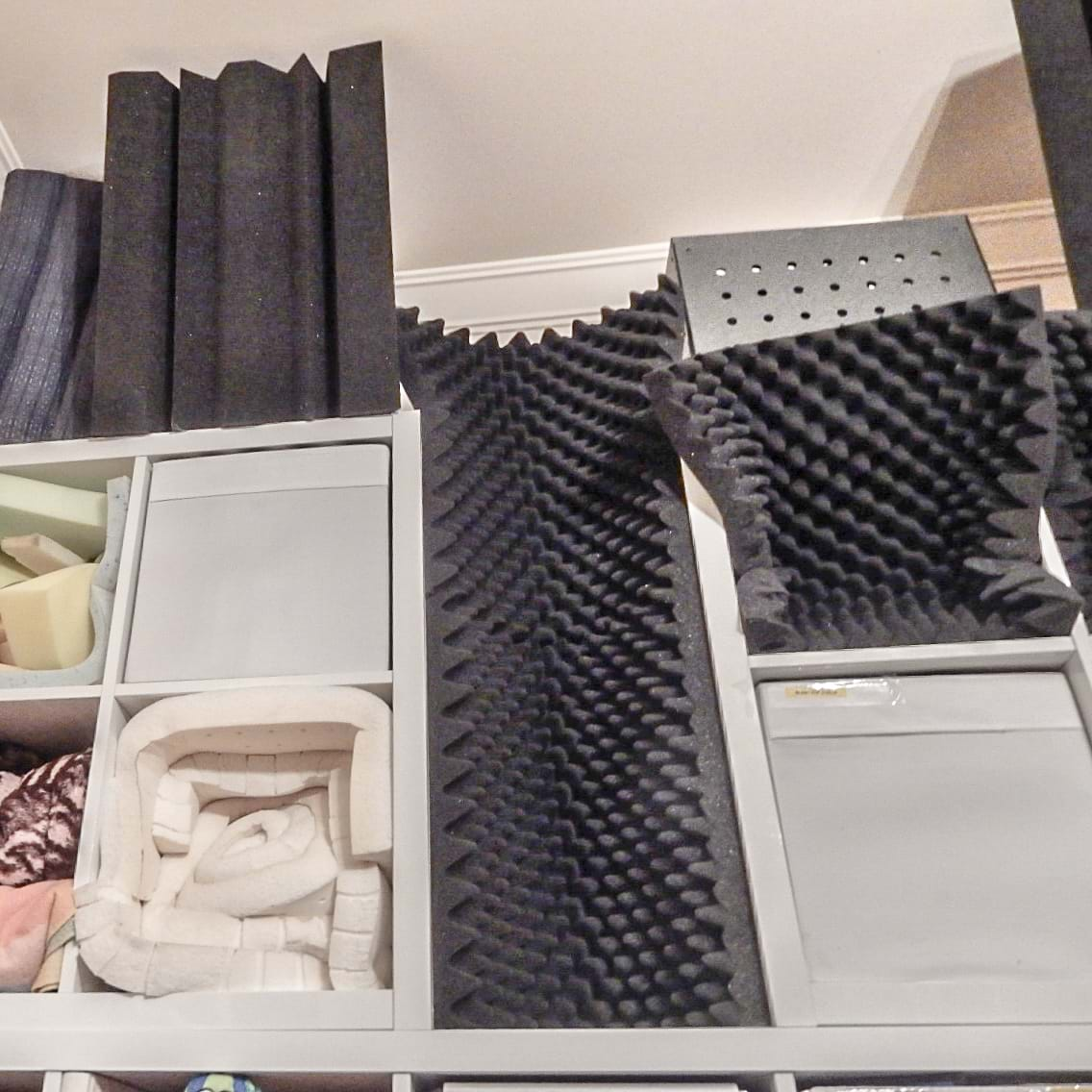
Dictionary.com. Lexico Publishing Group, LLC. Accessed December 19, 2005. (Source of some vocabulary definitions, with some adaptation.) http://www.dictionary.com
Contributors
Supporting program, acknowledgements.
The contents of this digital library curriculum were developed under grants from the Fund for the Improvement of Postsecondary Education (FIPSE), U.S. Department of Education and National Science Foundation (GK-12 grant no. 0338326). However, these contents do not necessarily represent the policies of the Department of Education or National Science Foundation, and you should not assume endorsement by the federal government.
Last modified: March 17, 2021
NOTIFICATIONS
Sound – visualising sound waves.
- + Create new collection
Sound is a form of energy that is caused by the vibration of matter. Sound is transmitted through waves, which travel through solids, liquids and gases. We are most used to the sound travelling through air, but sound is able to travel faster and further in solids and liquids .
‘Seeing’ sound
If we could see the molecules that make up the air around us, we would see sound as a series of more and less dense areas of air that are moving away from the source of the sound at about 340 metres per second. We say sound is a wave because the air molecules move back and forth while the sound travels along. The air behaves much like a longitudinal or compression wave on a spring.
It is difficult to draw compression waves , so waves are generally represented as transverse waves for simplicity. The dense areas of the compression wave are the peaks of the transverse wave and the sparse areas are the troughs.
Microphone – transforming sound energy into electrical energy
In order to visualise a sound wave, we can use a microphone to transform sound energy into electrical energy. A simple microphone is made up of a very thin membrane with a coil of very fine wire attached. A magnet is positioned so that it is just inside the coil of wire but not touching it. When a sound wave strikes the membrane , it jiggles (vibrates) back and forth because of the high and low pressure areas of the wave. This causes the coil to jiggle, and when a coil moves in a magnetic field, an electrical current is produced. If we look at the electrical current using an oscilloscope, we can see the sound as a series of peaks and troughs.
The sound from single pitch or note will make a simple sine wave on the screen. The wave will change as you change the volume or pitch of the note.
Related content
This article is part of an article series :
- Sound – understanding standing waves
- Sound – resonance
- Sound – wave interference
- Sound – beats, the Doppler effect and sonic booms
with the accompanying investigations:
- Measuring the speed of sound
- Investigating sound wave resonance
Additional articles and activity ideas
Find out more about studying sound under water and read about what is needed for sound to be heard , and how sound travels through water to understand some of the key science concepts.
Investigating waves and energy uses slinkies to explore longitudinal and transverse waves.
Make and use a hydrophone explains how to construct an underwater microphone.
Sound on an oscilloscope uses a computer’s microphone to create a visual display.
The PLD article Physical World – Sound curates Hub resources for the early years through to year 10.
Visit the sound topic for additional resources.
See our newsletters here .
Would you like to take a short survey?
This survey will open in a new tab and you can fill it out after your visit to the site.
- Random article
- Teaching guide
- Privacy & cookies
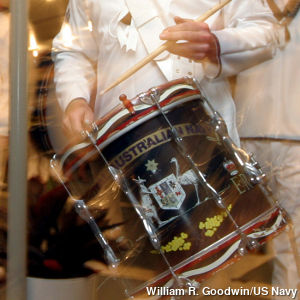
by Chris Woodford . Last updated: July 23, 2023.
Photo: Sound is energy we hear made by things that vibrate. Photo by William R. Goodwin courtesy of US Navy and Wikimedia Commons .
What is sound?
Photo: Sensing with sound: Light doesn't travel well through ocean water: over half the light falling on the sea surface is absorbed within the first meter of water; 100m down and only 1 percent of the surface light remains. That's largely why mighty creatures of the deep rely on sound for communication and navigation. Whales, famously, "talk" to one another across entire ocean basins, while dolphins use sound, like bats, for echolocation. Photo by Bill Thompson courtesy of US Fish and Wildlife Service .
Robert Boyle's classic experiment
Artwork: Robert Boyle's famous experiment with an alarm clock.
How sound travels
Artwork: Sound waves and ocean waves compared. Top: Sound waves are longitudinal waves: the air moves back and forth along the same line as the wave travels, making alternate patterns of compressions and rarefactions. Bottom: Ocean waves are transverse waves: the water moves back and forth at right angles to the line in which the wave travels.
The science of sound waves
Picture: Reflected sound is extremely useful for "seeing" underwater where light doesn't really travel—that's the basic idea behind sonar. Here's a side-scan sonar (reflected sound) image of a World War II boat wrecked on the seabed. Photo courtesy of U.S. National Oceanographic and Atmospheric Administration, US Navy, and Wikimedia Commons .
Whispering galleries and amphitheaters
Photos by Carol M. Highsmith: 1) The Capitol in Washington, DC has a whispering gallery inside its dome. Photo credit: The George F. Landegger Collection of District of Columbia Photographs in Carol M. Highsmith's America, Library of Congress , Prints and Photographs Division. 2) It's easy to hear people talking in the curved memorial amphitheater building at Arlington National Cemetery, Arlington, Virginia. Photo credit: Photographs in the Carol M. Highsmith Archive, Library of Congress , Prints and Photographs Division.

Measuring waves
Understanding amplitude and frequency, why instruments sound different, the speed of sound.
Photo: Breaking through the sound barrier creates a sonic boom. The mist you can see, which is called a condensation cloud, isn't necessarily caused by an aircraft flying supersonic: it can occur at lower speeds too. It happens because moist air condenses due to the shock waves created by the plane. You might expect the plane to compress the air as it slices through. But the shock waves it generates alternately expand and contract the air, producing both compressions and rarefactions. The rarefactions cause very low pressure and it's these that make moisture in the air condense, producing the cloud you see here. Photo by John Gay courtesy of US Navy and Wikimedia Commons .
Why does sound go faster in some things than in others?
Chart: Generally, sound travels faster in solids (right) than in liquids (middle) or gases (left)... but there are exceptions!
How to measure the speed of sound
Sound in practice, if you liked this article..., find out more, on this website.
- Electric guitars
- Speech synthesis
- Synthesizers
On other sites
- Explore Sound : A comprehensive educational site from the Acoustical Society of America, with activities for students of all ages.
- Sound Waves : A great collection of interactive science lessons from the University of Salford, which explains what sound waves are and the different ways in which they behave.
Educational books for younger readers
- Sound (Science in a Flash) by Georgia Amson-Bradshaw. Franklin Watts/Hachette, 2020. Simple facts, experiments, and quizzes fill this book; the visually exciting design will appeal to reluctant readers. Also for ages 7–9.
- Sound by Angela Royston. Raintree, 2017. A basic introduction to sound and musical sounds, including simple activities. Ages 7–9.
- Experimenting with Sound Science Projects by Robert Gardner. Enslow Publishers, 2013. A comprehensive 120-page introduction, running through the science of sound in some detail, with plenty of hands-on projects and activities (including welcome coverage of how to run controlled experiments using the scientific method). Ages 9–12.
- Cool Science: Experiments with Sound and Hearing by Chris Woodford. Gareth Stevens Inc, 2010. One of my own books, this is a short introduction to sound through practical activities, for ages 9–12.
- Adventures in Sound with Max Axiom, Super Scientist by Emily Sohn. Capstone, 2007. The original, graphic novel (comic book) format should appeal to reluctant readers. Ages 8–10.
Popular science
- The Sound Book: The Science of the Sonic Wonders of the World by Trevor Cox. W. W. Norton, 2014. An entertaining tour through everyday sound science.
Academic books
- Master Handbook of Acoustics by F. Alton Everest and Ken Pohlmann. McGraw-Hill Education, 2015. A comprehensive reference for undergraduates and sound-design professionals.
- The Science of Sound by Thomas D. Rossing, Paul A. Wheeler, and F. Richard Moore. Pearson, 2013. One of the most popular general undergraduate texts.
Text copyright © Chris Woodford 2009, 2021. All rights reserved. Full copyright notice and terms of use .
Rate this page
Tell your friends, cite this page, more to explore on our website....
- Get the book
- Send feedback
Want to create or adapt books like this? Learn more about how Pressbooks supports open publishing practices.
Traveling waves
17 How sound moves
Speed of sound.
There’s a delay between when a sound is created and when it is heard. In everyday life, the delay is usually too short to notice. However, the delay can be noticeable if the distance between source and detector is large enough. You see lightning before you hear the thunder. If you’ve sat in the outfield seats in a baseball stadium, you’ve experienced the delay between seeing the player hit the ball and the sound of the “whack.” Life experiences tell us that sound travels fast, but not nearly as fast as light does. Careful experiments confirm this idea.
The speed of sound in air is roughly 340 m/s. The actual value depends somewhat on the temperature and humidity. In everyday terms, sound travels about the length of three and a half foot ball fields every second- about 50% faster than a Boeing 747 (roughly 250 m/s). This may seem fast, but it’s tiny compared to light, which travels roughly a million times faster than sound (roughly 300,000,000 m/s).
Sound requires some material in which to propagate (i.e. travel). This material sound travels through is called the medium . You can show that sound requires a medium by putting a cell phone inside a glass jar connected to a vacuum pump. As the air is removed from the jar, the cell phone’s ringer gets quieter and quieter. A youTube video (2:05 min) produced by the UNSW PhysClips project shows the demo with a drumming toy monkey [1] instead of a cell phone.
What affects the speed of sound?
Sound travels at different speeds though different materials. The physical properties of the medium are the only factors that affect the speed of sound- nothing else matters.
The speed of sound in a material is determined mainly by two properties- the stiffness of the material and the density of the material. Sound travels fastest through materials that are stiff and light. In general, sound travels fastest through solids, slower through liquids and slowest through gasses. (See the table on this page). This may seem backwards- after all, metals are quite dense. However, the high density of metals is more than offset by far greater stiffness (compared to liquids and solids).
The speed of sound in air depends mainly on temperature. The speed of sound is 331 m/s in dry air at 0 o Celsius and increases slightly with temperature- about 0.6 m/s for every 1 o Celsius for temperatures commonly found on Earth. Though speed of sound in air also depends on humidity, the effect is tiny- sound travels only about 1 m/s faster in air with 100% humidity air at 20 o C than it does in completely dry air at the same temperature.
Nothing else matters
The properties of the medium are the only factors that affect the speed of sound- nothing else matters.
Frequency of the sound does not matter- high frequency sounds travel at the same speed as low frequency sounds. If you’ve ever listened to music, you’ve witnessed this- the low notes and the high notes that were made simultaneously reach you simultaneously, even if you are far from the stage. If you’ve heard someone shout from across a field, you’ve noticed that the entire shout sound (which contains many different frequencies at once) reaches you at the same time. If different frequencies traveled at different rates, some frequencies would arrive before others.
The amplitude of the sound does not matter- loud sounds and quiet ones travel at the same speed. Whisper or yell- it doesn’t matter. The sound still takes the same amount of time to reach the listener. You’ve probably heard that you can figure out how far away the lightning by counting the seconds between when you see lightning and hear thunder. If the speed of sound depended on loudness, this rule of thumb would have to account for loudness- yet there is nothing in the rule about loud vs. quiet thunder. The rule of thumb works the same for all thunder- regardless of loudness . That’s because the speed of sound doesn’t depend on amplitude.
Stop to thinks
- Which takes longer to cross a football field: the sound of a high pitched chirp emitted by a fruit bat or the (relatively) low pitched sound emitted by a trumpet?
- Which sound takes longer to travel 100 meters: the sound of a snapping twig in the forest or the sound of a gunshot?
- Which takes longer to travel the distance of a football field: the low pitched sound of a whale or the somewhat higher pitched sound of a human being?
Constant speed
Sound travels at a constant speed. Sound does not speed up or slow down as it travels (unless the properties of the material the sound is going through changes). I know what you’re thinking- how is that possible? Sounds die out as they travel, right? True. That means sounds must slow down and come to a stop, right? Wrong. As sound travels, its amplitude decreases- but that’s not the same thing as slowing down. Sound (in air) covers roughly 340 meters each and every second, even as its amplitude shrinks. Eventually, the amplitude gets small enough that the sound is undetectable. A sound’s amplitude shrinks as it travels, but its speed remains constant.
The basic equation for constant speed motion (shown below) applies to sound.
[latex]d=vt[/latex]
In this equation, [latex]d[/latex] represents the distance traveled by the sound, [latex]t[/latex] represents the amount of time it took to go that distance and [latex]v[/latex] represents the speed.
Rule of thumb for lightning example
Example: thunder and lightning.
The rule of thumb for figuring out how far away a lightning strike is from you is this:
Count the number of seconds between when you see the lightning and hear the thunder. Divide the number of seconds by five to find out how many miles away the lightning hit.
According to this rule, what is the speed of sound in air? How does the speed of sound implied by this rule compare to 340 m/s?
Identify important physics concept : This question is about speed of sound.
List known and unknown quantities (with letter names and units):
At first glance, it doesn’t look like there’s enough information to solve the problem. We were asked to find speed, but not given either a time or a distance. However, the problem does allow us to figure out a distance if we know the time- “Divide the number of seconds by five to find out how many miles away the lightning hit.” So, let’s make up a time and see what happens; if the time is 10 seconds, the rule of thumb says that the distance should be 2 miles.
[latex]v= \: ?[/latex]
[latex]d=2 \: miles[/latex]
[latex]t=10 \: seconds[/latex]
You might ask “Is making stuff up OK here?” The answer is YES! If the rule of thumb is right, it should work no matter what time we choose. (To check if the rule is OK, we should probably test it with more than just one distance-time combination, but we’ll assume the rule is OK for now).
Do the algebra: The equation is already solved for speed. Move on to the next step.
Do unit conversions (if needed) then plug in numbers: If you just plug in the numbers, the speed comes out in miles per second:
[latex]v = \frac{2 \: miles} {10 \: seconds}=0.2 \: \frac{miles} {second}[/latex]
We are asked to compare this speed to 340 m/s, so a unit conversion is in order; since there are 1609 meters in a mile:
[latex]v =0.2 \: \frac{miles} {second}*\frac{1609 \: meters} {1 \:mile}=320 \frac{m}{s}[/latex]
Reflect on the answer:
- The answer for speed from the rule of thumb is less than 10% off the actual value of roughly 340 m/s- surprisingly close!
- At the beginning, we assumed a time of 10 seconds. Does the result hold up for other choices? A quick check shows that it does! For instance, if we use a time of 5 seconds, the rule of thumb gives a distance of 1 mile, and the math still gives a speed of 0.2 miles/second. The speed will be the same no matter what time we pick. The reason is this: The more time it takes the thunder to arrive, the farther away the lightning strike is, but the speed remains the same. In the equation for speed, both time and distance change by the same factor and the overall fraction remains unchanged.
Stop to think answers
- Both sounds take the same amount of time. (High and low pitched sounds travel at the same speed).
- Both sounds take the same amount of time. (Quiet sounds and loud sounds travel at the same speed).
- The sound of the whale travels the distance in less time- assuming sound from the whale travels in water and sound from the human travels in air. Sound travels faster in water than in air. (The info about frequency was given just to throw you off- frequency doesn’t matter).
- Wolfe, J. (2014, February 20). Properties of Sound. Retrieved from https://www.youtube.com/watch?v=P8-govgAffY ↵
Understanding Sound Copyright © by dsa2gamba and abbottds is licensed under a Creative Commons Attribution 4.0 International License , except where otherwise noted.
Share This Book
Every Degree Counts: Unravelling the Mysteries of Temperature.
- Culinary & Beverages
- Weather and Climate
- Technology & Engineering
- Home, Lifestyle & DIY
- Science & Environment
- Health & Nutrition
- Art & Crafts
- Outdoor & Recreation
- Fashion & Beauty
Education & Research
Home > Education & Research > The Speed Of Sound: Comparing Solid, Liquid, And Gas
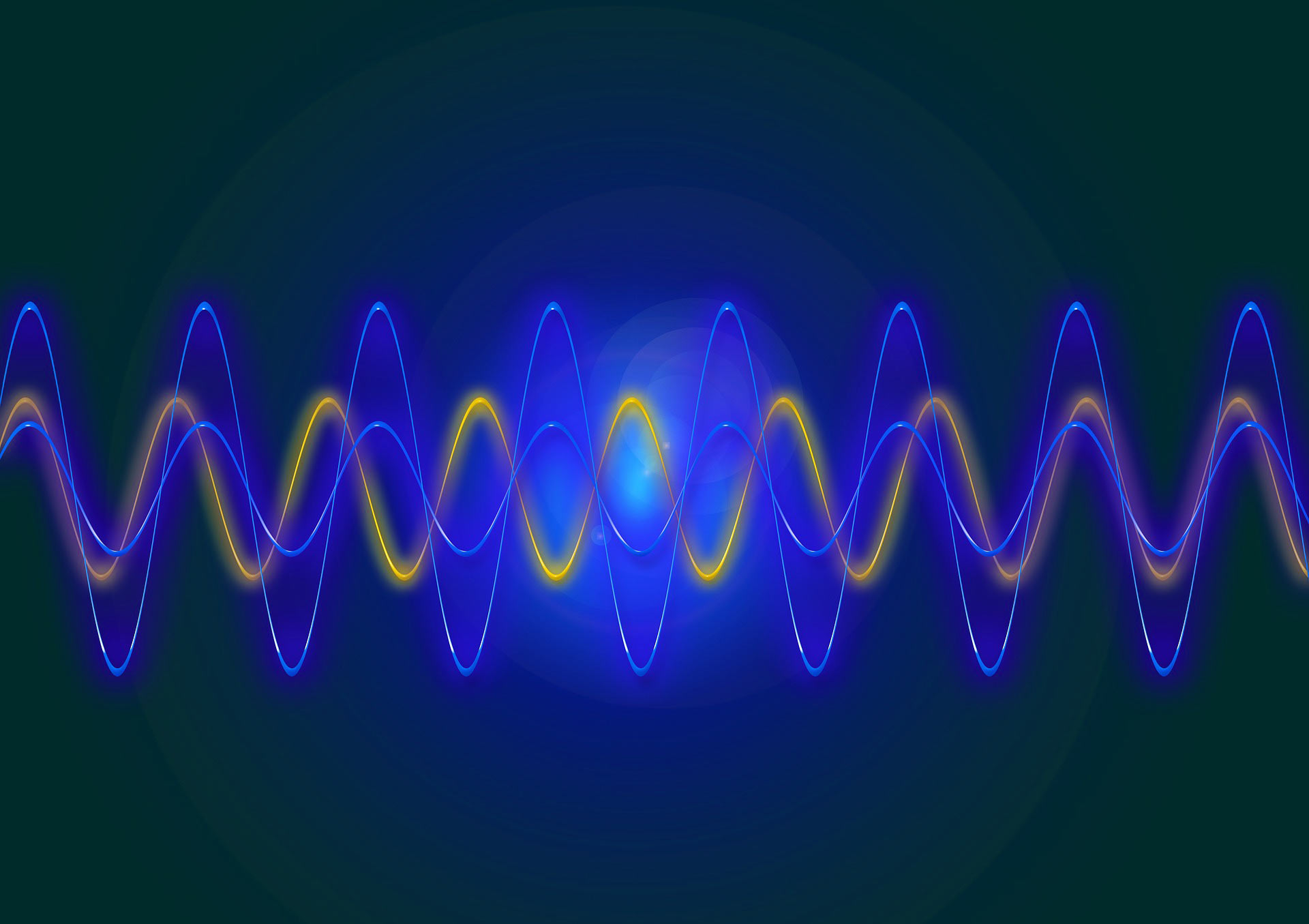
The Speed Of Sound: Comparing Solid, Liquid, And Gas
Published: February 19, 2024
Written by: Kyle Russell
Explore the speed of sound in solid, liquid, and gas with our educational research. Learn about the fascinating properties of different states of matter.
(Many of the links in this article redirect to a specific reviewed product. Your purchase of these products through affiliate links helps to generate commission for Temperatures.com, at no extra cost. Learn more )
Table of Contents
Introduction, properties of solid, liquid, and gas, speed of sound in solids, speed of sound in liquids, speed of sound in gases, factors affecting the speed of sound in different states of matter.
The speed of sound is a fascinating phenomenon that varies across different states of matter. In solids, liquids, and gases, the speed of sound exhibits distinct characteristics, influenced by the unique properties of each state. Understanding these differences not only enriches our knowledge of physics but also provides valuable insights into the behavior of materials under varying conditions.
In this article, we will delve into the intriguing realm of acoustics, exploring the speed of sound in solids, liquids, and gases. By examining the properties and behaviors of these states of matter, we can unravel the underlying factors that contribute to the transmission of sound waves. From the tightly packed particles of solids to the free-flowing molecules of gases, each state presents a distinct environment through which sound travels.
Join us on this captivating journey as we uncover the intricacies of sound propagation in different states of matter, shedding light on the fundamental principles that govern the speed of sound. Let's embark on this enlightening exploration, where the realms of physics and material science converge to unveil the mysteries of acoustic velocity.
Read more : The Temperature Of Liquid Nitrogen
- In solids, particles are densely packed in a regular, three-dimensional arrangement, resulting in a fixed shape and volume. The strong intermolecular forces hold the particles in a relatively stable position, allowing only vibrational motion. This rigidity gives solids their characteristic resistance to deformation and high density.
- Liquids exhibit a moderate level of particle arrangement, with particles maintaining close proximity but possessing more freedom of movement compared to solids. This allows liquids to take the shape of their container while maintaining a constant volume. The intermolecular forces in liquids are weaker than those in solids, enabling particles to flow past each other, giving liquids their fluidity.
- Gases have the most disordered arrangement of particles, with large intermolecular spaces and high kinetic energy. This results in gases having neither a fixed shape nor volume, as they expand to fill the available space. The weak intermolecular forces in gases allow for rapid and random motion of particles, leading to their compressibility and low density.
Understanding the distinct properties of solids, liquids, and gases provides a foundation for comprehending the behavior of sound waves in these different states of matter. The varying degrees of particle arrangement and intermolecular forces in each state significantly influence the transmission of sound, contributing to the diverse speeds of sound propagation observed in solids, liquids, and gases.
Read more : Comparing Spontaneous And Nonspontaneous Phenomena
In solids, the transmission of sound waves occurs through the propagation of mechanical vibrations. These vibrations travel as longitudinal waves, where particles oscillate back and forth in the direction of wave propagation. The speed of sound in solids is influenced by the elastic properties of the material, particularly its bulk modulus and density.
The bulk modulus represents the material's resistance to compression, determining how the particles respond to applied forces. Solids with higher bulk moduli exhibit stiffer behavior, leading to faster sound propagation. Additionally, the density of the solid plays a crucial role in determining the speed of sound. Denser materials, with particles closely packed together, facilitate faster transmission of sound waves due to the shorter distances between particles.
The atomic structure of solids also contributes to the speed of sound. In crystalline solids, where particles are arranged in a regular and repeating pattern, sound waves can travel more efficiently compared to amorphous solids, which lack a defined crystalline structure. This is attributed to the orderly arrangement of particles in crystalline solids, allowing for more coherent transmission of mechanical vibrations.
Furthermore, the presence of defects or imperfections in the solid's structure can affect the speed of sound. Dislocations, vacancies, and grain boundaries can impede the smooth propagation of sound waves, leading to variations in the speed of sound within the material.
An interesting phenomenon observed in solids is the anisotropy of sound propagation. Some crystalline materials exhibit different speeds of sound along different crystallographic directions. This anisotropic behavior arises from the asymmetry in the atomic arrangement within the crystal lattice, resulting in varying elastic properties along different axes.
In summary, the speed of sound in solids is intricately linked to the material's elastic properties, density, atomic structure, and the presence of defects. These factors collectively contribute to the unique characteristics of sound transmission in solids, showcasing the remarkable interplay between material science and acoustics.
In the realm of liquids, the propagation of sound waves unfolds within a distinctive environment characterized by the fluidity and intermolecular dynamics of the liquid medium. Unlike solids, where particles are tightly bound in a fixed lattice, and gases, where particles exhibit rapid and random motion, liquids strike a balance between cohesion and mobility, giving rise to intriguing behaviors in sound transmission.
The speed of sound in liquids is influenced by several key factors, with the bulk modulus and density of the liquid playing pivotal roles. The bulk modulus, representing the resistance to compression, governs the transmission of acoustic waves through the liquid medium. Liquids with higher bulk moduli exhibit greater resistance to compression, resulting in faster propagation of sound waves. Additionally, the density of the liquid contributes to the speed of sound, with denser liquids facilitating swifter transmission of acoustic energy due to the closer proximity of particles.
Furthermore, the intermolecular forces within the liquid medium significantly impact the speed of sound. Stronger intermolecular forces lead to a more cohesive and ordered arrangement of particles, promoting faster transmission of sound waves. Conversely, weaker intermolecular forces allow for more freedom of movement among particles, influencing the speed of sound propagation in liquids.
Viscosity, a measure of the internal friction within the liquid, also influences the speed of sound. Higher viscosity can impede the transmission of sound waves, leading to a decrease in the speed of sound in the liquid medium. Additionally, temperature plays a crucial role, as variations in temperature can alter the intermolecular interactions and density of the liquid, consequently affecting the speed of sound.
It is noteworthy that the speed of sound in liquids is generally higher than in gases but lower than in solids. This intermediate nature of sound propagation in liquids reflects the unique balance of intermolecular forces, density, and viscosity present in the liquid state.
In summary, the speed of sound in liquids is intricately linked to the cohesive forces, density, viscosity, and temperature of the liquid medium. These factors collectively shape the transmission of sound waves in liquids, offering a captivating glimpse into the nuanced interplay between material properties and acoustic phenomena.
In the realm of gases, the transmission of sound waves unfolds within a dynamic and intricate environment characterized by the rapid and random motion of gas particles. Unlike solids and liquids, gases lack a fixed structure and exhibit high compressibility and low density, setting the stage for unique behaviors in sound propagation.
The speed of sound in gases is primarily influenced by the elastic properties of the gas, particularly its adiabatic bulk modulus and density. The adiabatic bulk modulus represents the gas's resistance to compression under adiabatic conditions, reflecting the responsiveness of gas particles to changes in pressure. Gases with higher adiabatic bulk moduli transmit sound waves more rapidly due to their increased resistance to compression. Additionally, the density of the gas plays a crucial role in determining the speed of sound. Denser gases, with particles closer together, facilitate faster transmission of sound waves due to the reduced interparticle distances.
The molecular composition of gases also impacts the speed of sound. In diatomic gases, such as oxygen and nitrogen, the presence of strong covalent bonds between atoms contributes to higher speeds of sound compared to monatomic gases, such as helium and neon. This is attributed to the increased rigidity and responsiveness of diatomic gas molecules to acoustic disturbances, leading to swifter transmission of sound waves.
Temperature plays a significant role in shaping the speed of sound in gases. As temperature rises, the kinetic energy of gas particles increases, leading to more vigorous and rapid motion. This heightened molecular activity results in a corresponding increase in the speed of sound, as the acoustic disturbances propagate more swiftly through the gas medium. Conversely, lower temperatures lead to reduced molecular motion and a decrease in the speed of sound.
It is noteworthy that the speed of sound in gases is significantly lower than in solids and higher than in liquids. This intermediate nature of sound propagation in gases reflects the unique interplay between molecular dynamics, density, and temperature present in the gaseous state.
In summary, the speed of sound in gases is intricately linked to the adiabatic bulk modulus, density, molecular composition, and temperature of the gas medium. These factors collectively shape the transmission of sound waves in gases, offering a captivating glimpse into the nuanced interplay between material properties and acoustic phenomena.
The speed of sound in solids, liquids, and gases is influenced by a myriad of factors, reflecting the intricate interplay between material properties and acoustic phenomena. These factors shape the transmission of sound waves, giving rise to distinct behaviors in each state of matter.
Read more : The Low Temperatures On Gas Giants: Explained
Solid State
In solids, the speed of sound is profoundly impacted by the material's elastic properties, density, and atomic structure. The bulk modulus, representing the resistance to compression, governs the transmission of mechanical vibrations, with higher bulk moduli leading to faster sound propagation. Additionally, the density of the solid plays a crucial role, as denser materials facilitate swifter transmission of sound waves due to the shorter distances between particles. The atomic structure, particularly in crystalline solids, contributes to efficient sound transmission, while the presence of defects can impede the smooth propagation of sound waves, leading to variations in the speed of sound within the material.
Liquid State
In liquids, the speed of sound is influenced by the bulk modulus, density, intermolecular forces, viscosity, and temperature. The bulk modulus and density govern the transmission of acoustic waves, with stronger intermolecular forces and higher density contributing to faster sound propagation. Viscosity, a measure of internal friction, can impede the transmission of sound waves, leading to a decrease in the speed of sound. Temperature variations alter intermolecular interactions and density, consequently affecting the speed of sound in liquids.
Gaseous State
In gases, the speed of sound is primarily influenced by the adiabatic bulk modulus, density, molecular composition, and temperature. Gases with higher adiabatic bulk moduli transmit sound waves more rapidly, and the molecular composition impacts the speed of sound, with diatomic gases exhibiting higher speeds compared to monatomic gases. Temperature plays a significant role, as increased kinetic energy leads to swifter propagation of sound waves through the gas medium.
Understanding these factors provides valuable insights into the diverse behaviors of sound propagation in different states of matter, showcasing the remarkable interplay between material science and acoustics. The nuanced interplay between elastic properties, density, intermolecular forces, and temperature shapes the transmission of sound waves, offering a captivating glimpse into the fundamental principles that govern the speed of sound in solids, liquids, and gases.
In conclusion, the exploration of the speed of sound in solids, liquids, and gases unveils a captivating tapestry of material properties and acoustic phenomena. The distinct behaviors observed in each state of matter reflect the intricate interplay between particle arrangement, intermolecular forces, and temperature, shaping the transmission of sound waves in remarkable ways.
In solids, the speed of sound is intricately linked to the material's elastic properties, density, and atomic structure. The bulk modulus and density play pivotal roles in governing the transmission of mechanical vibrations, while the presence of defects and the crystalline nature of solids contribute to the diverse speeds of sound propagation observed within different materials.
Moving to liquids, the speed of sound is influenced by the cohesive forces, density, viscosity, and temperature of the liquid medium. The balance between intermolecular dynamics and fluidity gives rise to an intermediate speed of sound, reflecting the nuanced interplay between material properties and acoustic phenomena in the liquid state.
In the realm of gases, the speed of sound is primarily shaped by the adiabatic bulk modulus, density, molecular composition, and temperature. The rapid and random motion of gas particles, coupled with the responsiveness of the gas medium to changes in pressure and temperature, leads to unique behaviors in sound propagation, highlighting the dynamic nature of acoustic phenomena in the gaseous state.
By unraveling the factors that influence the speed of sound in solids, liquids, and gases, we gain a deeper appreciation for the fundamental principles that govern acoustics and material science. The diverse behaviors observed in sound propagation across different states of matter underscore the intricate relationship between material properties and the transmission of mechanical vibrations, offering a captivating glimpse into the profound interplay between physics and material science.
Ultimately, the exploration of the speed of sound in solids, liquids, and gases enriches our understanding of the fundamental principles that govern acoustics, providing valuable insights into the behavior of materials under varying conditions. This journey through the realms of physics and material science unveils the mysteries of acoustic velocity, shedding light on the remarkable interplay between material properties and the transmission of sound waves.
Was this page helpful?
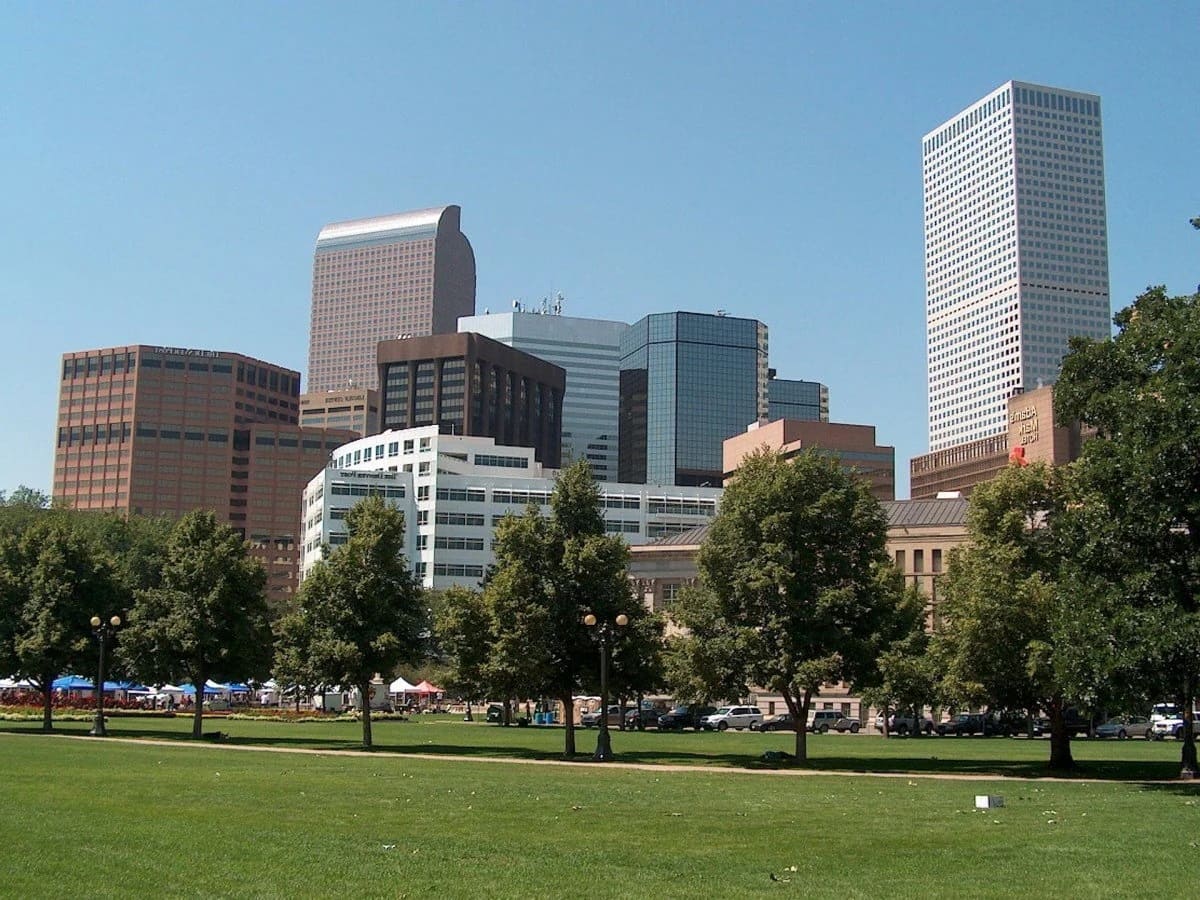
Denver Weather: A Comprehensive Guide To The Year-Round Climate In Denver
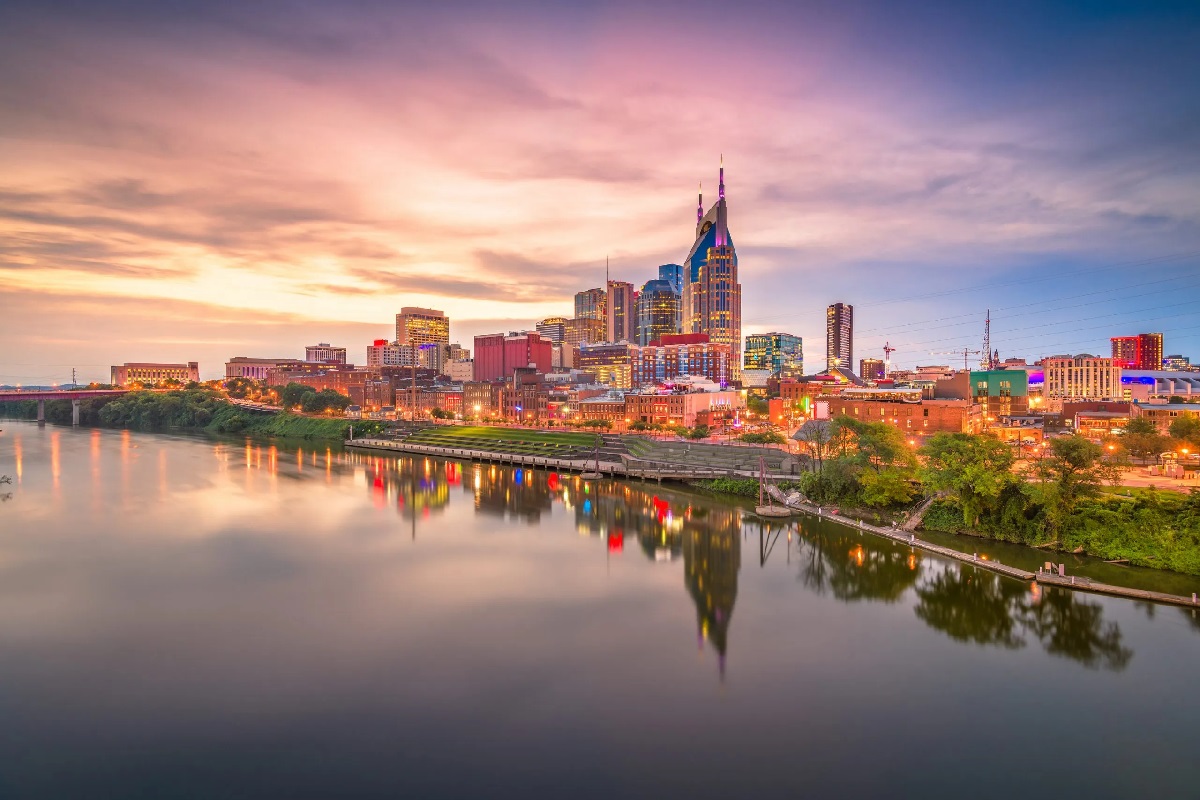
Nashville Weather: A Comprehensive Guide To Year-Round Climate
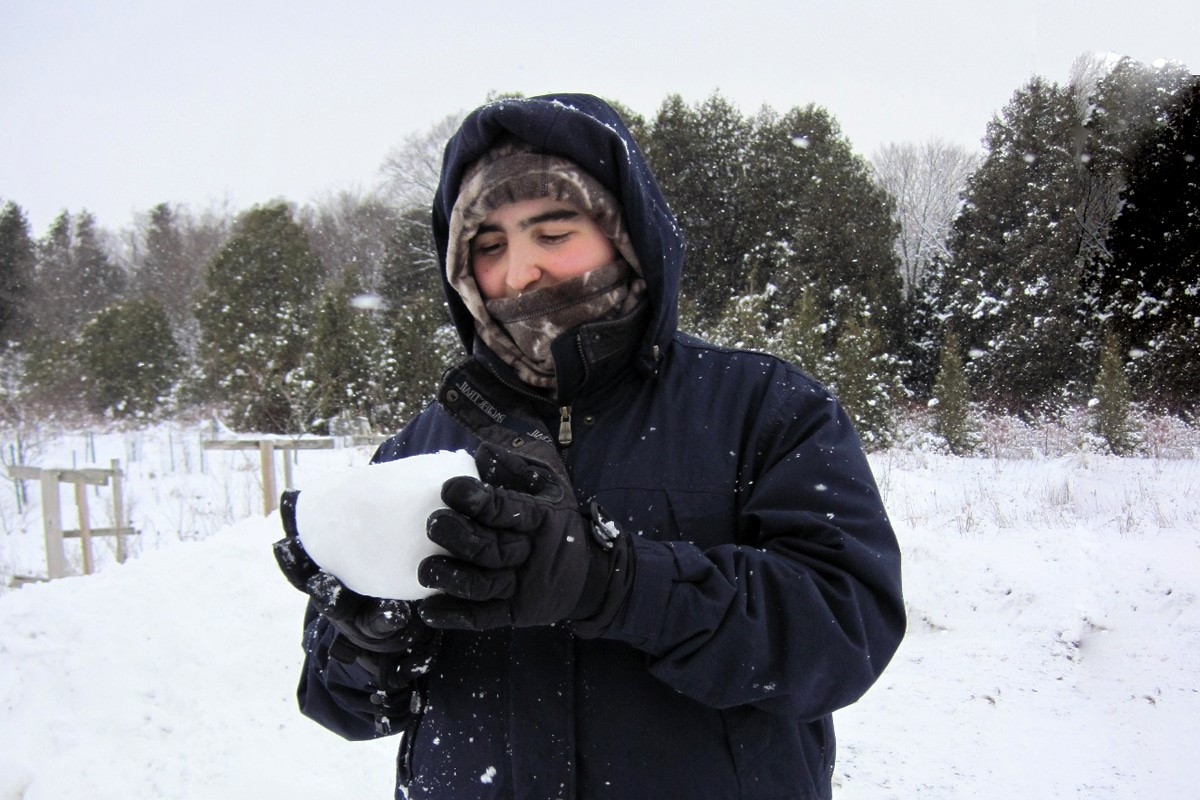
The Best Glue For Cold Weather
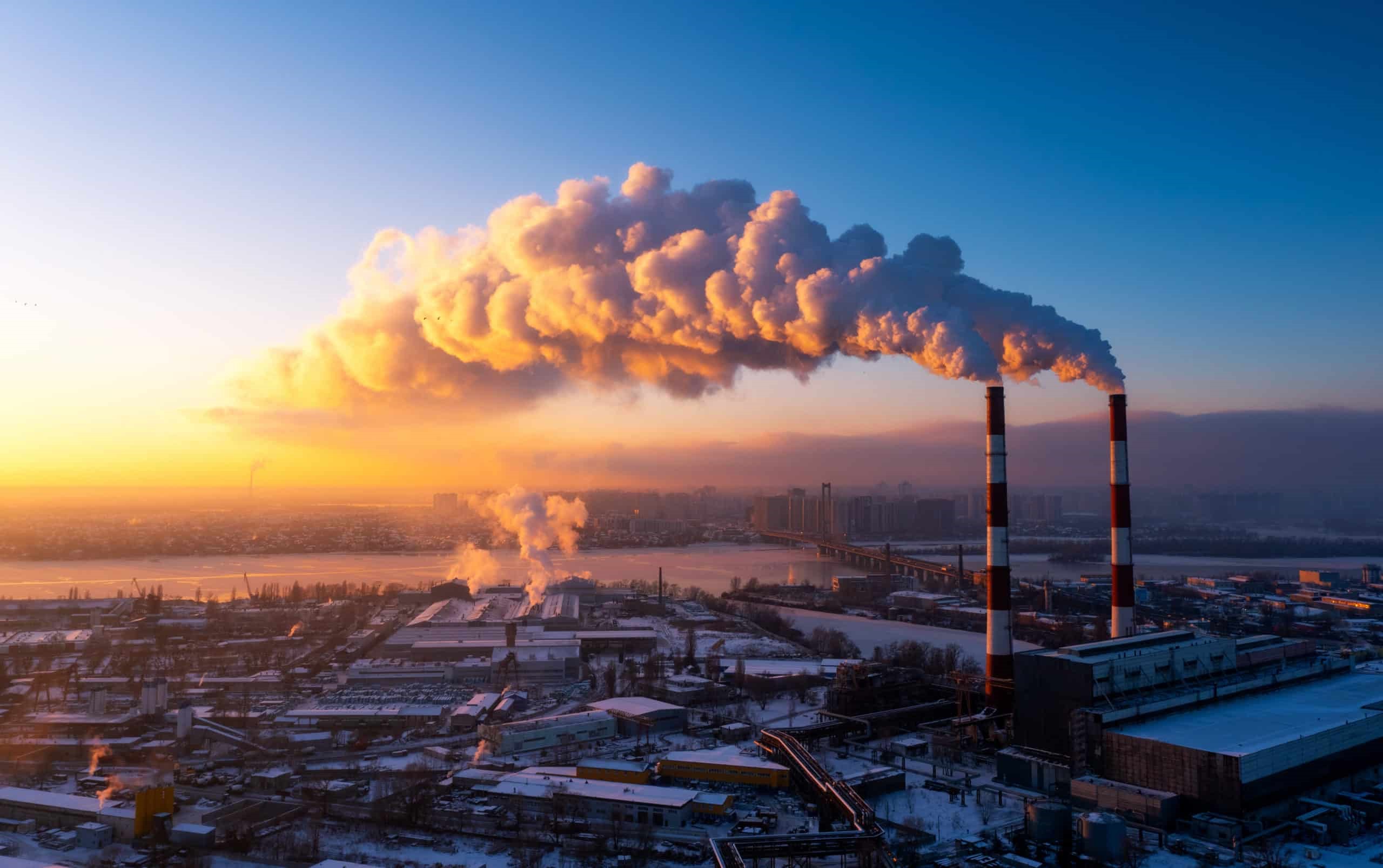
Understanding Greenhouse Gases: Causes, Effects, And Solutions
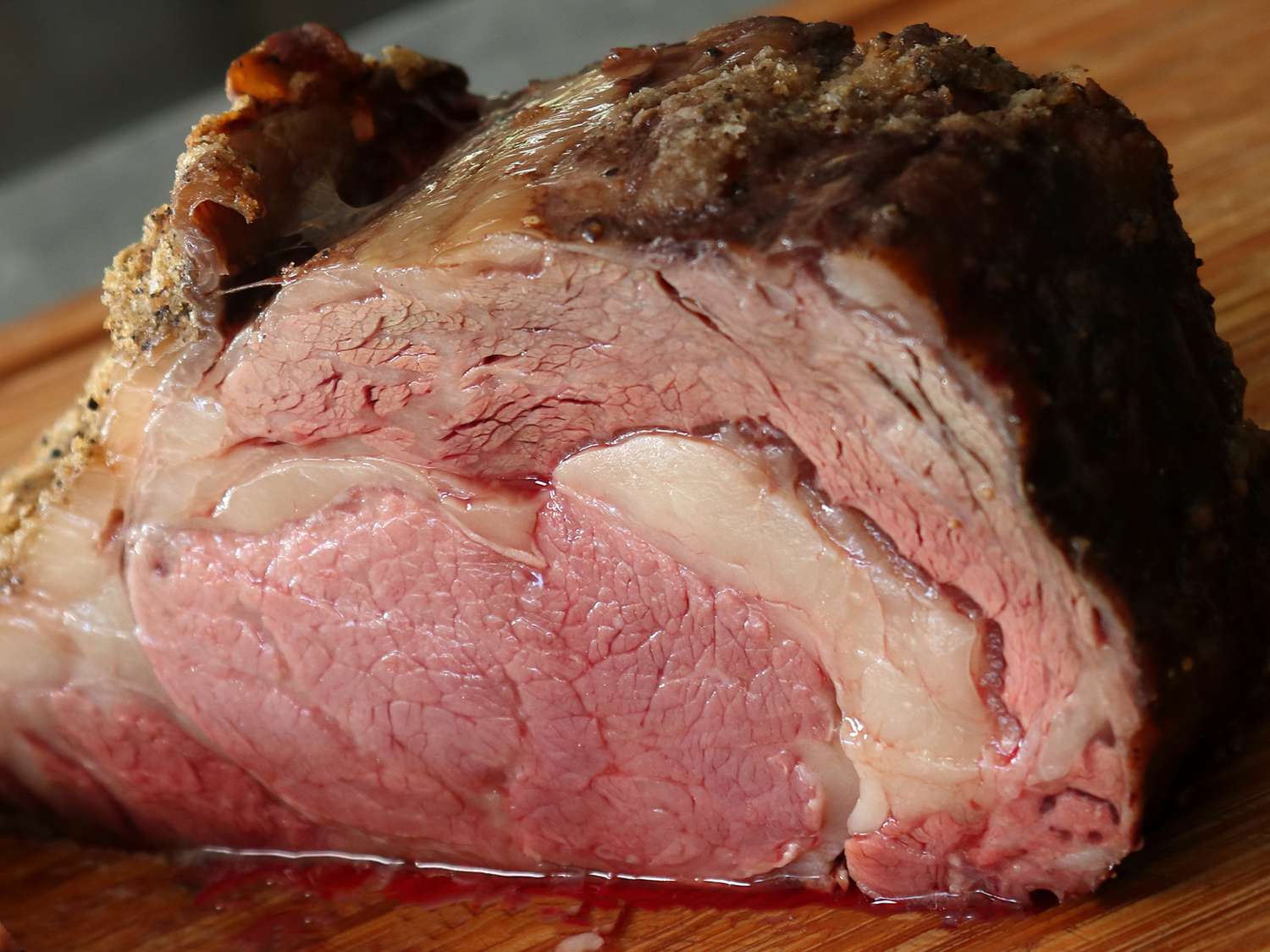
Perfect Temperature For Medium Rare Prime Rib
Latest articles.
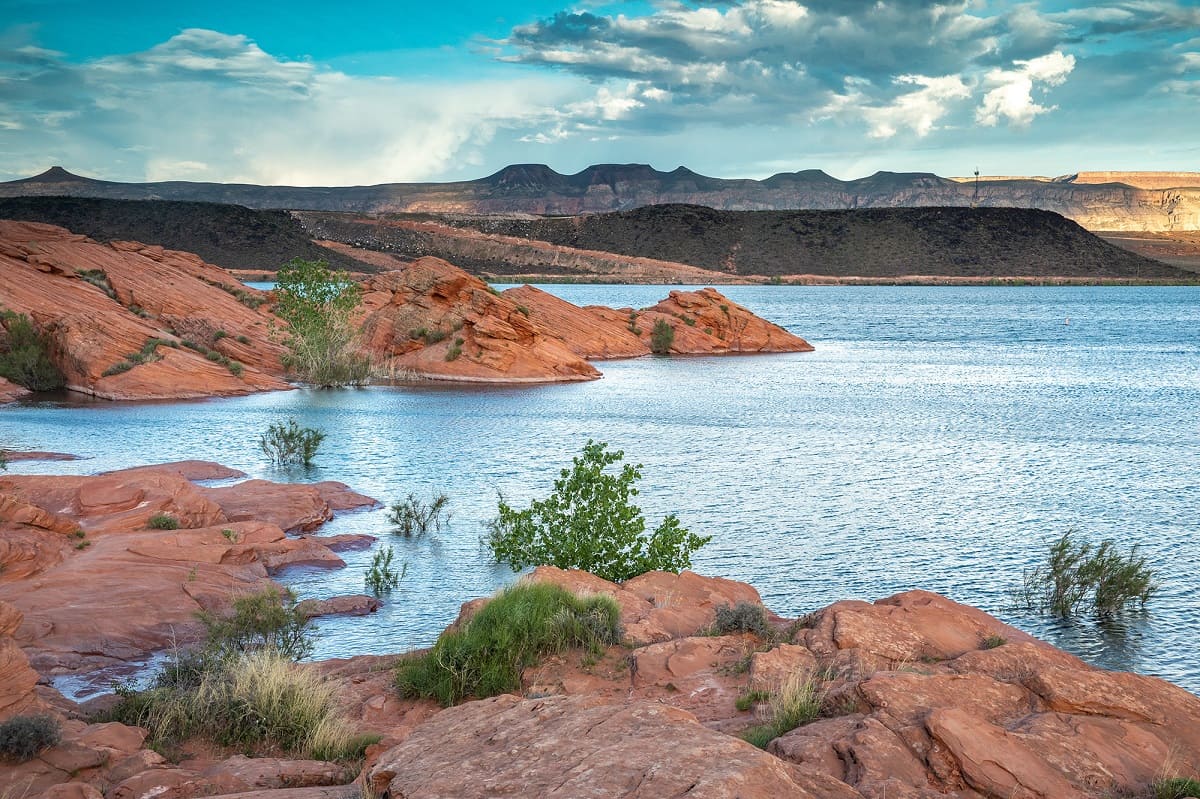
Understanding The Water Temperature Of Utah Lakes
Written By: Kyle Russell
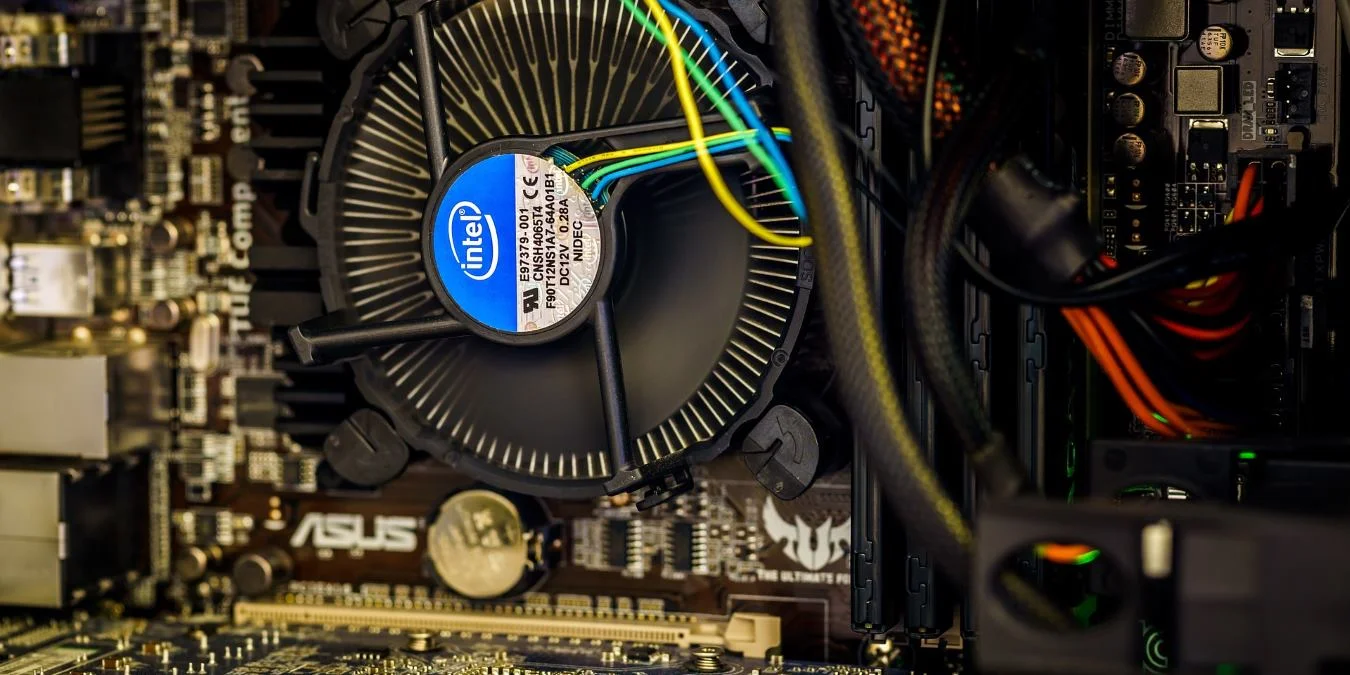
Optimizing CPU Temperature: Tips And Tricks For Better Performance
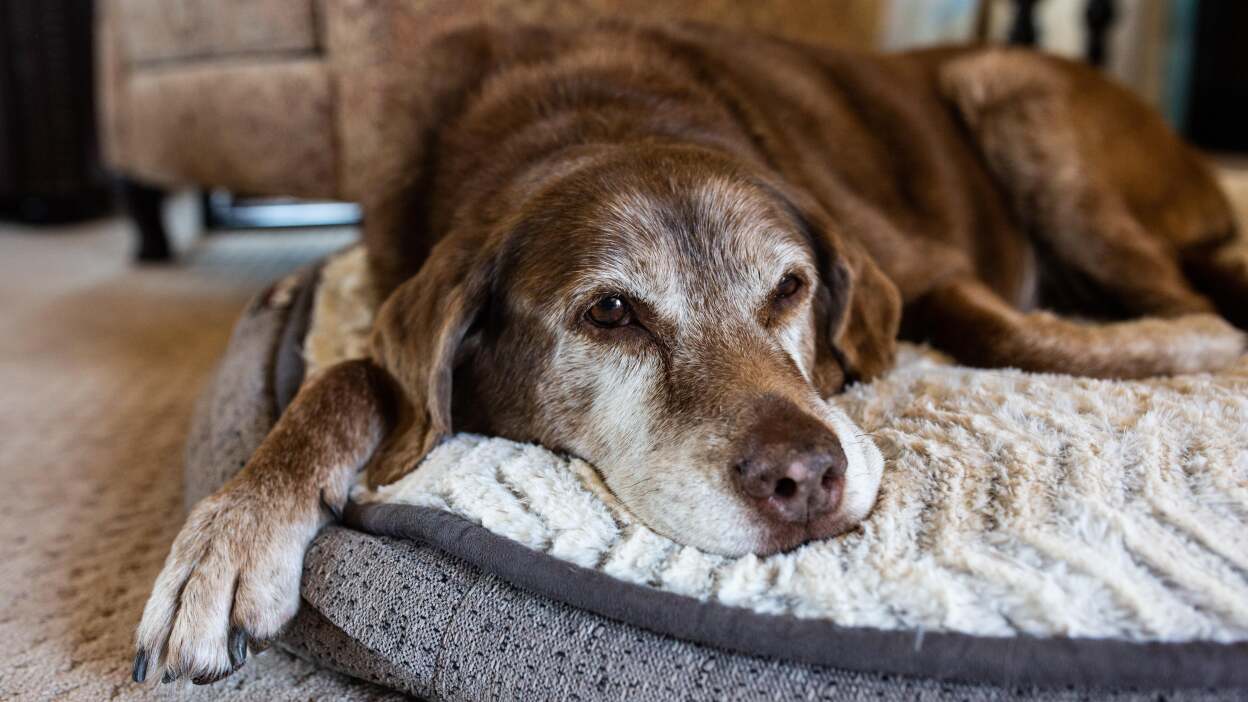
Ideal Indoor Temperature For Dogs: Ensuring A Comfortable Environment For Your Canine Companion
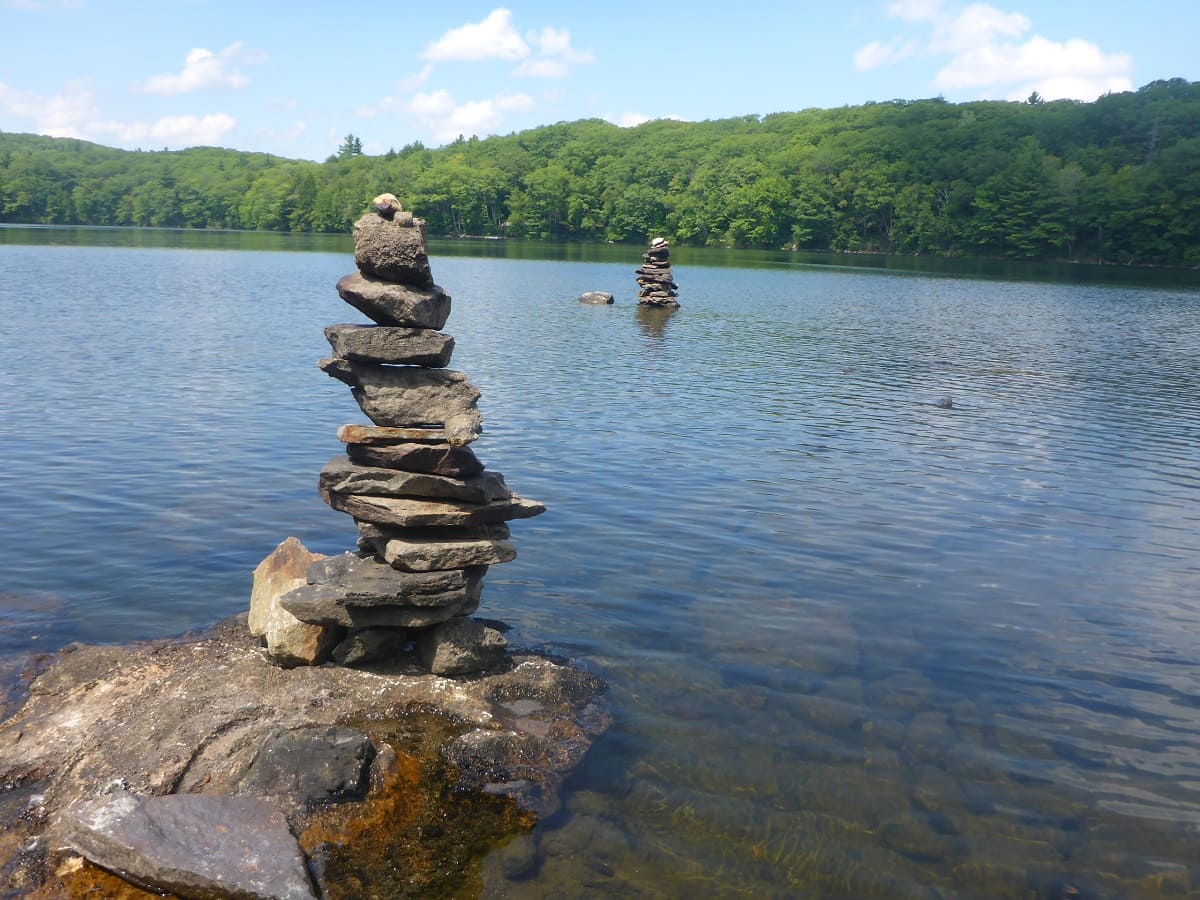
Average Freshwater Temperatures In North American Lakes
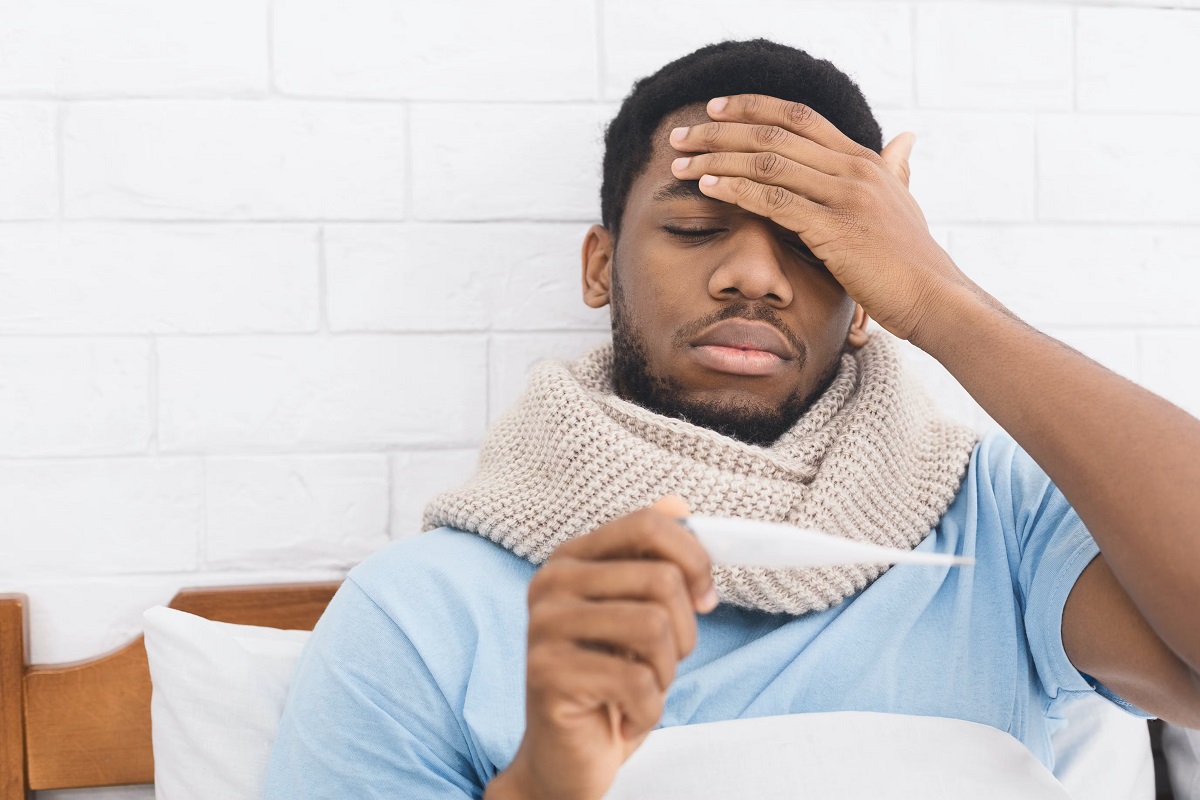
Understanding The Symptoms Of Fever
Related post.
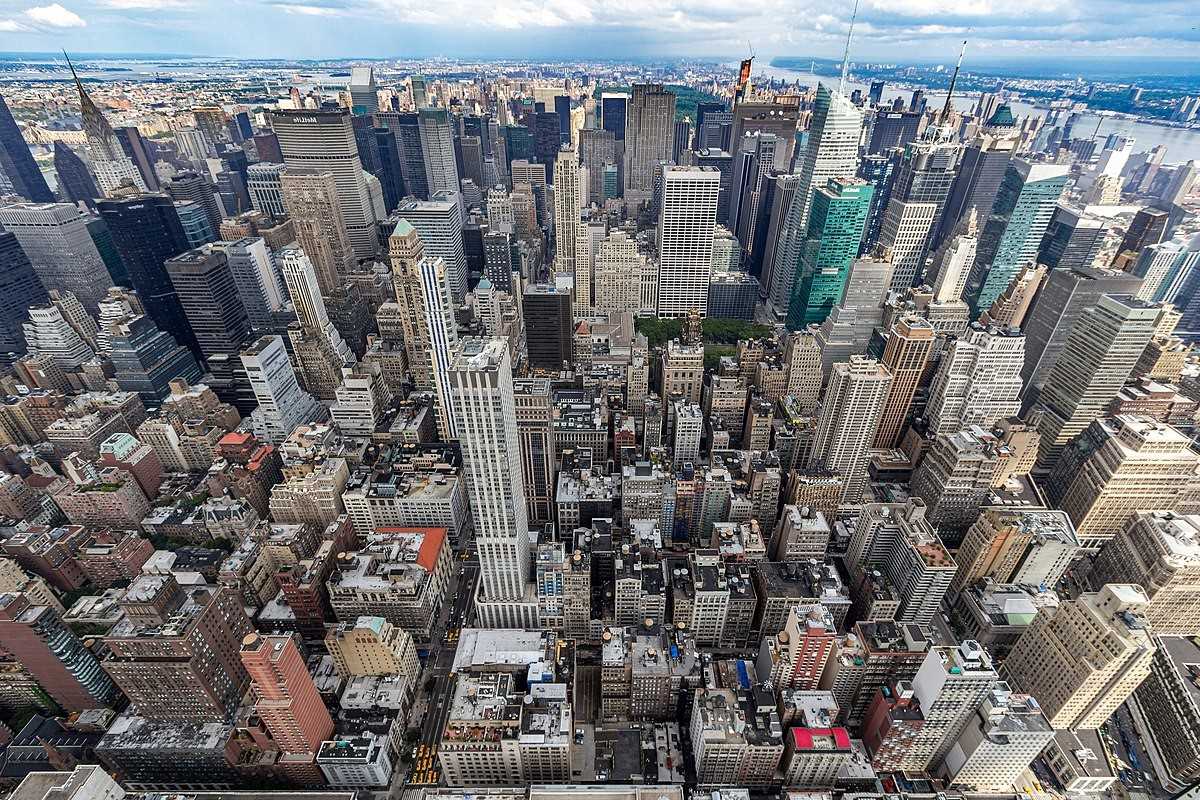
By: Kyle Russell • Education & Research
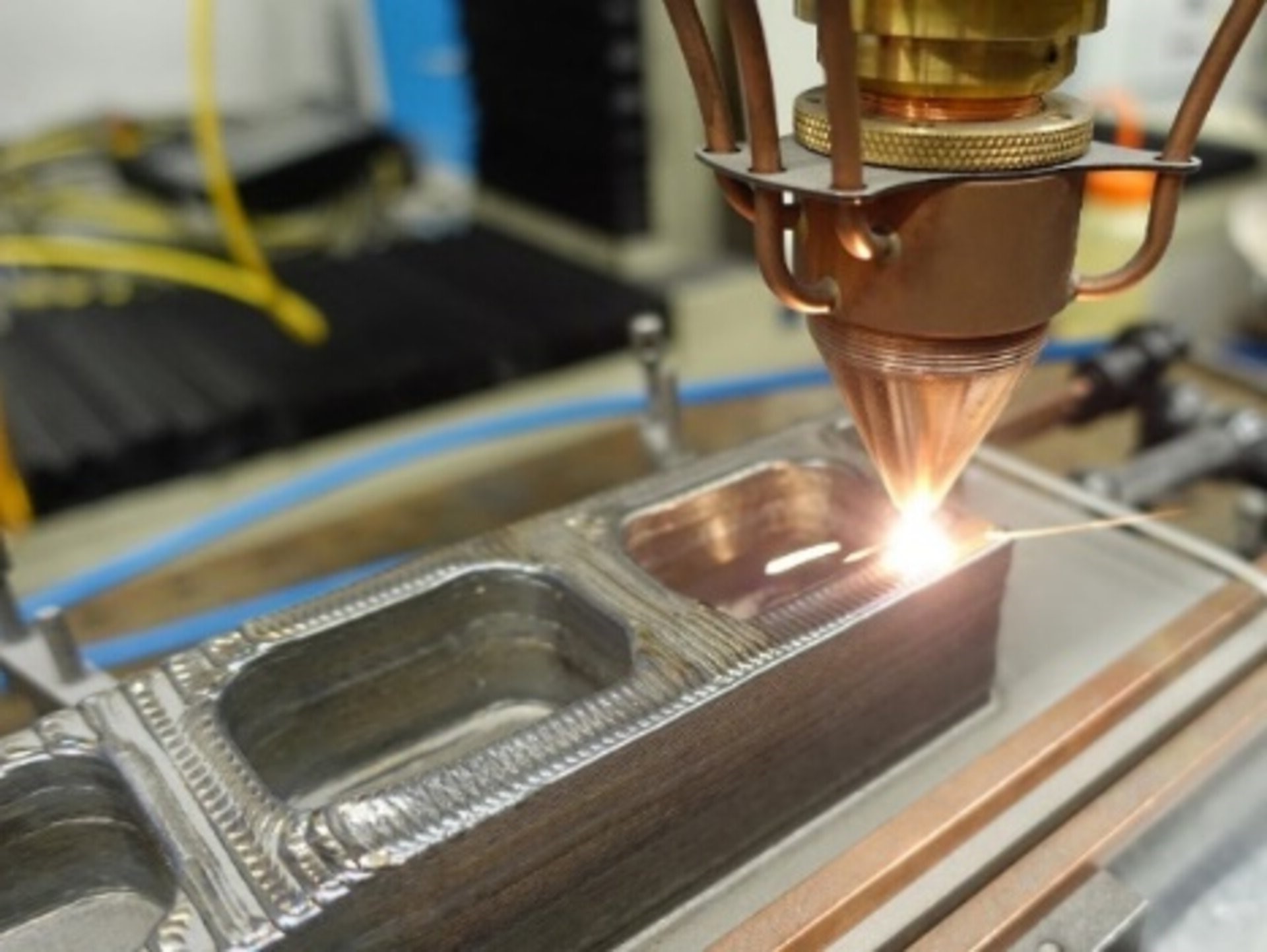
Please accept our Privacy Policy.
Temperatures.com uses cookies to improve your experience and to show you personalized ads. Please review our privacy policy by clicking here .
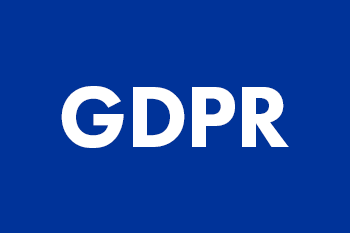
- Privacy Overview
- Strictly Necessary Cookies
This website uses cookies so that we can provide you with the best user experience possible. Cookie information is stored in your browser and performs functions such as recognising you when you return to our website and helping our team to understand which sections of the website you find most interesting and useful.
Strictly Necessary Cookie should be enabled at all times so that we can save your preferences for cookie settings.
If you disable this cookie, we will not be able to save your preferences. This means that every time you visit this website you will need to enable or disable cookies again.
- https://temperatures.com/education-research/the-speed-of-sound-comparing-solid-liquid-and-gas/
13.1 Types of Waves
Section learning objectives.
By the end of this section, you will be able to do the following:
- Define mechanical waves and medium, and relate the two
- Distinguish a pulse wave from a periodic wave
- Distinguish a longitudinal wave from a transverse wave and give examples of such waves
Teacher Support
The learning objectives in this section will help your students master the following standards:
- (A) examine and describe oscillatory motion and wave propagation in various types of media.
Section Key Terms
Mechanical waves.
What do we mean when we say something is a wave? A wave is a disturbance that travels or propagates from the place where it was created. Waves transfer energy from one place to another, but they do not necessarily transfer any mass. Light, sound, and waves in the ocean are common examples of waves. Sound and water waves are mechanical waves ; meaning, they require a medium to travel through. The medium may be a solid, a liquid, or a gas, and the speed of the wave depends on the material properties of the medium through which it is traveling. However, light is not a mechanical wave; it can travel through a vacuum such as the empty parts of outer space.
A familiar wave that you can easily imagine is the water wave. For water waves, the disturbance is in the surface of the water, an example of which is the disturbance created by a rock thrown into a pond or by a swimmer splashing the water surface repeatedly. For sound waves, the disturbance is caused by a change in air pressure, an example of which is when the oscillating cone inside a speaker creates a disturbance. For earthquakes, there are several types of disturbances, which include the disturbance of Earth’s surface itself and the pressure disturbances under the surface. Even radio waves are most easily understood using an analogy with water waves. Because water waves are common and visible, visualizing water waves may help you in studying other types of waves, especially those that are not visible.
Water waves have characteristics common to all waves, such as amplitude , period , frequency , and energy , which we will discuss in the next section.
Misconception Alert
Many people think that water waves push water from one direction to another. In reality, however, the particles of water tend to stay in one location only, except for moving up and down due to the energy in the wave. The energy moves forward through the water, but the water particles stay in one place. If you feel yourself being pushed in an ocean, what you feel is the energy of the wave, not the rush of water. If you put a cork in water that has waves, you will see that the water mostly moves it up and down.
[BL] [OL] [AL] Ask students to give examples of mechanical and nonmechanical waves.
Pulse Waves and Periodic Waves
If you drop a pebble into the water, only a few waves may be generated before the disturbance dies down, whereas in a wave pool, the waves are continuous. A pulse wave is a sudden disturbance in which only one wave or a few waves are generated, such as in the example of the pebble. Thunder and explosions also create pulse waves. A periodic wave repeats the same oscillation for several cycles, such as in the case of the wave pool, and is associated with simple harmonic motion. Each particle in the medium experiences simple harmonic motion in periodic waves by moving back and forth periodically through the same positions.
[BL] Any kind of wave, whether mechanical or nonmechanical, or transverse or longitudinal, can be in the form of a pulse wave or a periodic wave.
Consider the simplified water wave in Figure 13.2 . This wave is an up-and-down disturbance of the water surface, characterized by a sine wave pattern. The uppermost position is called the crest and the lowest is the trough . It causes a seagull to move up and down in simple harmonic motion as the wave crests and troughs pass under the bird.
Longitudinal Waves and Transverse Waves
Mechanical waves are categorized by their type of motion and fall into any of two categories: transverse or longitudinal. Note that both transverse and longitudinal waves can be periodic. A transverse wave propagates so that the disturbance is perpendicular to the direction of propagation. An example of a transverse wave is shown in Figure 13.3 , where a woman moves a toy spring up and down, generating waves that propagate away from herself in the horizontal direction while disturbing the toy spring in the vertical direction.
In contrast, in a longitudinal wave , the disturbance is parallel to the direction of propagation. Figure 13.4 shows an example of a longitudinal wave, where the woman now creates a disturbance in the horizontal direction—which is the same direction as the wave propagation—by stretching and then compressing the toy spring.
Tips For Success
Longitudinal waves are sometimes called compression waves or compressional waves , and transverse waves are sometimes called shear waves .
Teacher Demonstration
Transverse and longitudinal waves may be demonstrated in the class using a spring or a toy spring, as shown in the figures.
Waves may be transverse, longitudinal, or a combination of the two . The waves on the strings of musical instruments are transverse (as shown in Figure 13.5 ), and so are electromagnetic waves, such as visible light. Sound waves in air and water are longitudinal. Their disturbances are periodic variations in pressure that are transmitted in fluids.
Sound in solids can be both longitudinal and transverse. Essentially, water waves are also a combination of transverse and longitudinal components, although the simplified water wave illustrated in Figure 13.2 does not show the longitudinal motion of the bird.
Earthquake waves under Earth’s surface have both longitudinal and transverse components as well. The longitudinal waves in an earthquake are called pressure or P-waves, and the transverse waves are called shear or S-waves. These components have important individual characteristics; for example, they propagate at different speeds. Earthquakes also have surface waves that are similar to surface waves on water.
Energy propagates differently in transverse and longitudinal waves. It is important to know the type of the wave in which energy is propagating to understand how it may affect the materials around it.
Watch Physics
Introduction to waves.
This video explains wave propagation in terms of momentum using an example of a wave moving along a rope. It also covers the differences between transverse and longitudinal waves, and between pulse and periodic waves.
- After a compression wave, some molecules move forward temporarily.
- After a compression wave, some molecules move backward temporarily.
- After a compression wave, some molecules move upward temporarily.
- After a compression wave, some molecules move downward temporarily.
Fun In Physics
The physics of surfing.
Many people enjoy surfing in the ocean. For some surfers, the bigger the wave, the better. In one area off the coast of central California, waves can reach heights of up to 50 feet in certain times of the year ( Figure 13.6 ).
How do waves reach such extreme heights? Other than unusual causes, such as when earthquakes produce tsunami waves, most huge waves are caused simply by interactions between the wind and the surface of the water. The wind pushes up against the surface of the water and transfers energy to the water in the process. The stronger the wind, the more energy transferred. As waves start to form, a larger surface area becomes in contact with the wind, and even more energy is transferred from the wind to the water, thus creating higher waves. Intense storms create the fastest winds, kicking up massive waves that travel out from the origin of the storm. Longer-lasting storms and those storms that affect a larger area of the ocean create the biggest waves since they transfer more energy. The cycle of the tides from the Moon’s gravitational pull also plays a small role in creating waves.
Actual ocean waves are more complicated than the idealized model of the simple transverse wave with a perfect sinusoidal shape. Ocean waves are examples of orbital progressive waves , where water particles at the surface follow a circular path from the crest to the trough of the passing wave, then cycle back again to their original position. This cycle repeats with each passing wave.
As waves reach shore, the water depth decreases and the energy of the wave is compressed into a smaller volume. This creates higher waves—an effect known as shoaling .
Since the water particles along the surface move from the crest to the trough, surfers hitch a ride on the cascading water, gliding along the surface. If ocean waves work exactly like the idealized transverse waves, surfing would be much less exciting as it would simply involve standing on a board that bobs up and down in place, just like the seagull in the previous figure.
Additional information and illustrations about the scientific principles behind surfing can be found in the “Using Science to Surf Better!” video.
- The surfer would move side-to-side/back-and-forth vertically with no horizontal motion.
- The surfer would forward and backward horizontally with no vertical motion.
Check Your Understanding
Use these questions to assess students’ achievement of the section’s Learning Objectives. If students are struggling with a specific objective, these questions will help identify such objective and direct them to the relevant content.
- A wave is a force that propagates from the place where it was created.
- A wave is a disturbance that propagates from the place where it was created.
- A wave is matter that provides volume to an object.
- A wave is matter that provides mass to an object.
- No, electromagnetic waves do not require any medium to propagate.
- No, mechanical waves do not require any medium to propagate.
- Yes, both mechanical and electromagnetic waves require a medium to propagate.
- Yes, all transverse waves require a medium to travel.
- A pulse wave is a sudden disturbance with only one wave generated.
- A pulse wave is a sudden disturbance with only one or a few waves generated.
- A pulse wave is a gradual disturbance with only one or a few waves generated.
- A pulse wave is a gradual disturbance with only one wave generated.
What are the categories of mechanical waves based on the type of motion?
- Both transverse and longitudinal waves
- Only longitudinal waves
- Only transverse waves
- Only surface waves
In which direction do the particles of the medium oscillate in a transverse wave?
- Perpendicular to the direction of propagation of the transverse wave
- Parallel to the direction of propagation of the transverse wave
As an Amazon Associate we earn from qualifying purchases.
This book may not be used in the training of large language models or otherwise be ingested into large language models or generative AI offerings without OpenStax's permission.
Want to cite, share, or modify this book? This book uses the Creative Commons Attribution License and you must attribute Texas Education Agency (TEA). The original material is available at: https://www.texasgateway.org/book/tea-physics . Changes were made to the original material, including updates to art, structure, and other content updates.
Access for free at https://openstax.org/books/physics/pages/1-introduction
- Authors: Paul Peter Urone, Roger Hinrichs
- Publisher/website: OpenStax
- Book title: Physics
- Publication date: Mar 26, 2020
- Location: Houston, Texas
- Book URL: https://openstax.org/books/physics/pages/1-introduction
- Section URL: https://openstax.org/books/physics/pages/13-1-types-of-waves
© Jan 19, 2024 Texas Education Agency (TEA). The OpenStax name, OpenStax logo, OpenStax book covers, OpenStax CNX name, and OpenStax CNX logo are not subject to the Creative Commons license and may not be reproduced without the prior and express written consent of Rice University.
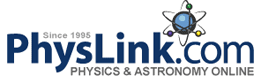
Shop Our Online Science Store
Solar & battery fan diy stem kit.
- $9.99 $5.95
Doodling Shake Bot DIY STEM Kit
- $8.99 $4.95
Hand-Crank Generator DIY STEM Kit
3-in-1 alternative energy car diy stem kit.
- $19.99 $14.95
Simple DC Motor DIY STEM Kit
- $7.99 $4.95
Flashing LED Circuit DIY Electronics Kit
- $4.99 $2.59
Wire Maze Electricity DIY STEM Kit
Fan micro car diy stem kit.
- $9.99 $4.95
- Privacy Policy
- Cookie Policy

IMAGES
VIDEO
COMMENTS
After solids, liquids have the highest speed of sound. And then finally gas, that included our air since it is made up of gasses. When a sound is traveling through one medium like air and then encounters another, like a solid door, it loses some of its energy and some of the volume will be lost.
The speed of sound at 20 degrees Celsius is about 343 meters per second, but the speed of sound at zero degrees Celsius is only about 331 meters per second. Remember, the only way to change the speed of sound is to change the properties of the medium it's travelling in and the speed of sound is typically faster through solids than it is through ...
The speed of sound is greatest for solids, intermediate for liquids, and lowest for gases: v solid > v liquid >v gas. Particles in a gas undergo elastic collisions and the particles are widely separated. In contrast, particles in a solid are locked into place (rigid or stiff), so a vibration readily transmits through chemical bonds. Here are ...
The velocity relation looks like: vsound in fluid = B ρ−−√ (2.1.1) (2.1.1) v s o u n d i n f l u i d = B ρ. Sound will also travel through a solid, but in that case the interactions of the particles are different than in a fluid, and the constant that takes the place of tension is a different one: Young's modulus. But the formula ...
Speed of sound is the speed at which sound waves move through the mediums like gas, liquid, solid and vacuum. The speed of sound in dry air is 343 m/s. Login. Study Materials. ... In general, sound travels faster in liquids than in gases and quicker in solids than in liquids. The greater the elasticity and the lower the density, the faster ...
Summary Students explore how sound waves move through liquids, solids and gases in a series of simple sound energy experiments. Understanding the properties of sound and how sound waves travel helps engineers determine the best room shape and construction materials when designing sound recording studios, classrooms, libraries, concert halls and theatres.
sound can travel through solids, liquids and gases sound cannot travel through a vacuum - it needs a medium to travel through the speed of sound in air is approximately 340 m/s
Sound - visualising sound waves. Sound is a form of energy that is caused by the vibration of matter. Sound is transmitted through waves, which travel through solids, liquids and gases. We are most used to the sound travelling through air, but sound is able to travel faster and further in solids and liquids.
The speed of sound is dependent on the properties of the material it travels through: it will travel faster through a solid than a liquid, and faster through a liquid than a gas. The speed of sound is the distance traveled in a unit of time by a sound wave through an elastic medium. This medium can be a solid, liquid, gas, or even plasma.
In non-humid air at 20 degrees Celsius, the speed of sound is about 343 meters per second or 767 miles per hour. We can also watch the speed of sound of a repeating simple harmonic wave. The speed of the wave can again be determined by the speed of the compressed regions as they travel through the medium.
In a gas or liquid, sound consists of compression waves. In solids, waves propagate as two different types. A longitudinal wave is associated with compression and decompression in the direction of travel, and is the same process in gases and liquids, with an analogous compression-type wave in solids. Only compression waves are supported in ...
Sound is caused by vibrations. These vibrations travel as waves through any medium: Sound can travel through gas, liquid and solids. sound travels best through solids as the particles are so close ...
Sound waves travel through the air as squashed-up compressions and stretched-out rarefactions. They only look like this on an oscilloscope trace. ... Sound travels at different speeds in solids, liquids, and gases. It's generally faster in solids than in liquids and faster in liquids than in gases: for example, ...
The speed of sound in a material is determined mainly by two properties- the stiffness of the material and the density of the material. Sound travels fastest through materials that are stiff and light. In general, sound travels fastest through solids, slower through liquids and slowest through gasses. (See the table on this page).
From the tightly packed particles of solids to the free-flowing molecules of gases, each state presents a distinct environment through which sound travels. Join us on this captivating journey as we uncover the intricacies of sound propagation in different states of matter, shedding light on the fundamental principles that govern the speed of sound.
Video transcript. Learn for free about math, art, computer programming, economics, physics, chemistry, biology, medicine, finance, history, and more. Khan Academy is a nonprofit with the mission of providing a free, world-class education for anyone, anywhere.
The speed of sound is different in different media. Sound travels with different speeds in different media like solid, liquid and gases. Sound travels in a m...
Sound travels at different speeds depending on what it is traveling through. Of the three mediums (gas, liquid, and solid) sound waves travel the slowest thr...
Cover one ear with your hand and the other ear with the bag of air. Have an assisstant tap the bag with a pencil. How does it sound? Now fill the bag with water (a liquid) and seal it. Hold this water-filled bag against one ear while covering the other ear with your hand. Have your assistant tap this bag with a pencil.
The experiment showed that Solid was the best of the 3 mediums for the sound to travel the loudest. Liquid medium was in the second place. Air medium gave the worst result. The results can be explained as follows. Sound travels as a wave of mechanical vibrations through mediums. Molecules in solids are closer to each other compared to liquid ...
Sound is made when things or air vibrate. Can sound travel through three states of matter? Find out in this video.
Light, sound, and waves in the ocean are common examples of waves. Sound and water waves are mechanical waves; meaning, they require a medium to travel through. The medium may be a solid, a liquid, or a gas, and the speed of the wave depends on the material properties of the medium through which it is traveling.
The reason why the sound velocity is usually larger in solids than in liquids and usually larger in liquids than in gases is because of the elastics constants of the material. What determines the elastic constants of a material is the interatomic bond strength. The stronger the bond, the higher the elastic constants.